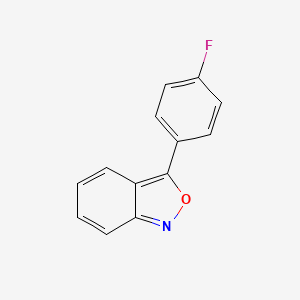
3-(4-Fluorophenyl)-2,1-benzisoxazole
Overview
Description
3-(4-Fluorophenyl)-2,1-benzisoxazole is a heterocyclic compound featuring a benzisoxazole core substituted at the 3-position with a 4-fluorophenyl group. The benzisoxazole scaffold is characterized by a fused benzene and isoxazole ring, which imparts rigidity and diverse electronic properties. Fluorine substitution on the phenyl ring enhances lipophilicity and metabolic stability, making this compound a candidate for pharmaceutical and materials science applications.
Scientific Research Applications
Medicinal Chemistry Applications
Anticancer Activity
Research indicates that 3-(4-Fluorophenyl)-2,1-benzisoxazole exhibits promising anticancer properties. In vitro studies have demonstrated its ability to inhibit the proliferation of various cancer cell lines by targeting specific signaling pathways involved in tumor growth. For instance, a study showed that derivatives of this compound could induce apoptosis in breast cancer cells through the activation of caspase pathways.
Antimicrobial Properties
The compound has also been evaluated for its antimicrobial activity. A series of tests against common bacterial strains revealed significant inhibitory effects on both Gram-positive and Gram-negative bacteria. Notably, Minimum Inhibitory Concentration (MIC) values ranged from 125 to 500 µg/mL against pathogens such as Staphylococcus aureus and Escherichia coli.
Microorganism | MIC (µg/mL) |
---|---|
Staphylococcus aureus | 125 |
Escherichia coli | 250 |
Pseudomonas aeruginosa | 500 |
Case Studies
-
Anticancer Mechanism Exploration
A study published in Journal of Medicinal Chemistry explored the structure-activity relationship of various benzisoxazole derivatives, including this compound. The study utilized molecular docking techniques to identify binding affinities to cancer-related targets such as protein kinases and transcription factors. The findings suggested that modifications to the fluorophenyl group significantly enhanced anticancer potency. -
Antimicrobial Evaluation
In a comprehensive evaluation published in Pharmaceutical Biology, researchers synthesized a library of benzisoxazole derivatives and tested their antimicrobial efficacy. The study concluded that the presence of the fluorine atom in the phenyl ring contributed to increased antibacterial activity, making these compounds potential candidates for developing new antibiotics.
Materials Science Applications
This compound has also found applications in materials science due to its unique electronic properties.
Organic Electronics
The compound is being investigated for its potential use in organic field-effect transistors (OFETs) and organic light-emitting diodes (OLEDs). Its π-conjugated structure allows for efficient charge transport, making it suitable for electronic applications. Preliminary studies indicate that films made from this compound exhibit good charge mobility and stability under operational conditions.
Biological Research Applications
In biological research, this compound serves as a valuable tool for studying enzyme interactions and cellular imaging.
Enzyme Inhibition Studies
Recent investigations have focused on the compound's ability to inhibit specific enzymes implicated in disease pathways. For example, studies demonstrated that it could effectively inhibit kinases involved in cancer progression, providing insights into its mechanism of action.
Fluorescent Probes
Due to its fluorescence properties, derivatives of this compound are being developed as fluorescent probes for cellular imaging. These probes allow researchers to visualize cellular processes in real-time, enhancing our understanding of cellular dynamics.
Q & A
Basic Research Questions
Q. What are the common synthetic routes for 3-(4-fluorophenyl)-2,1-benzisoxazole, and what are their yield optimization strategies?
- Methodology : Two primary methods are reported:
- Pd/Cu-Catalyzed Cross-Couplings : Suzuki-Miyaura coupling with aryl boronic acids yields derivatives (7–32% yields), while Sonogashira coupling with terminal alkynes gives 16–39% yields. Reaction conditions (e.g., solvent, catalyst loading) and purification (preparative MDF chromatography) are critical for purity (>90%) .
- Cyclization of Chalcones : Refluxing chalcone derivatives with hydroxylamine hydrochloride and K₂CO₃ in ethanol for 8 hours yields benzisoxazole derivatives. Crystallization from ethanol improves purity .
Q. How is structural characterization of this compound performed?
- Analytical Techniques :
- IR Spectroscopy : Key peaks include aromatic C-H stretch (~3225 cm⁻¹), C-F stretch (1324 cm⁻¹), and C=N stretch (1604 cm⁻¹) .
- ¹H NMR : Aromatic protons appear at δ 7.0–8.3 ppm, while olefinic protons (if present) resonate at δ 3.8–5.6 ppm .
- X-ray Crystallography : Used for analogous compounds (e.g., 3-(4-chlorophenyl)-2,1-benzisoxazole) to confirm bond angles and crystal packing .
Q. What in vitro assays are used to evaluate the biological activity of benzisoxazole derivatives?
- Anti-inflammatory Activity : Carrageenan-induced paw edema models in rodents are standard. Derivatives like [3-(4-fluorophenyl)ethenyl]-1,2-benzisoxazole show inhibition comparable to reference drugs (e.g., indomethacin) .
- Antibacterial Screening : Agar diffusion assays against Gram-positive (e.g., S. aureus) and Gram-negative (e.g., E. coli) strains, with MIC values reported .
Advanced Research Questions
Q. How can low yields in Sonogashira couplings of this compound be mitigated?
- Challenges : Sonogashira reactions with terminal alkynes often yield <20% due to side reactions (e.g., alkyne homocoupling).
- Solutions :
- Use Pd(PPh₃)₂Cl₂/CuI catalysts with polar solvents (DMF or THF) to stabilize intermediates.
- Add 2,6-lutidine to scavenge HCl, reducing side reactions .
- Data : Successful entries achieve 16–39% yields with >90% purity after MDF chromatography .
Q. What computational methods are used to predict the reactivity of this compound derivatives?
- DFT Studies : HOMO-LUMO gaps and Molecular Electrostatic Potential (MEP) maps identify nucleophilic/electrophilic sites. For example, the C-3 position is reactive toward electrophilic substitution .
- Molecular Dynamics (MD) : Simulate binding interactions with biological targets (e.g., COX-2 for anti-inflammatory activity) .
Q. How do structural modifications (e.g., fluorophenyl vs. piperidinyl substituents) affect biological activity?
- Case Study :
- 4-Fluorophenyl : Enhances lipophilicity, improving blood-brain barrier penetration in CNS-targeted compounds .
- Piperidinyl Substitution : Increases solubility and modulates receptor affinity (e.g., σ-receptor binding) .
- SAR Table :
Derivative | Substituent | IC₅₀ (Anti-inflammatory) | MIC (Antibacterial) |
---|---|---|---|
3-(4-Fluorophenyl) | -C₆H₄F | 12.5 μM | 8 μg/mL (S. aureus) |
6-Fluoro-3-piperidinyl | -N-piperidinyl | 18.3 μM | >32 μg/mL |
Q. How to resolve contradictions in reaction outcomes between similar benzisoxazole derivatives?
- Example : Hartwig-Buchwald coupling of 3-(2-fluoro-5-iodophenyl)benzisoxazole with alkyl hydrazines yields 14% via normal-phase chromatography but fails with MDF purification .
- Analysis :
- Purification Method : MDF may adsorb polar intermediates, reducing recovery. Normal-phase silica gel is preferable for polar hydrazine derivatives .
- Substrate Steric Effects : Bulkier substituents (e.g., 4,5-dimethoxyphenyl) hinder catalyst accessibility, lowering yields .
Q. Methodological Notes
- Synthetic Protocols : Prioritize Pd/Cu-catalyzed methods for diversification but optimize purification based on substituent polarity .
- Characterization : Combine NMR, IR, and X-ray data for unambiguous structural confirmation .
- Biological Assays : Include positive controls (e.g., indomethacin for inflammation) and validate results with triplicate experiments .
Comparison with Similar Compounds
B. Thermal Stability
- Electron-withdrawing substituents (e.g., fluorine at the 4-position) reduce thermal stability. For instance, 3-(4-Fluorophenyl)-2,1-benzisoxazole derivatives decompose near 30°C, limiting synthetic methods .
- Methylsulfanyl-substituted analogs (e.g., 8-methylsulfanyl-6,7-dihydrothieno[2,3-g]-2,1-benzisoxazole) exhibit higher thermal stability (synthesized in 59–70% yields) due to EDG effects .
A. MAO-B Inhibition
- p-Benzoitrile-substituted 2,1-benzisoxazoles (e.g., compound 7a ) show submicromolar MAO-B inhibition (IC₅₀ < 1 μM) via π-π stacking and van der Waals interactions .
- Chloro substituents at C5/C7 of the benzisoxazole ring enhance potency, while the fluorophenyl group in this compound may offer moderate activity due to its weaker electron-withdrawing effect compared to nitriles .
B. Anticonvulsant Activity
- Zonisamide (3-(sulfamoylmethyl)-1,2-benzisoxazole) demonstrates potent anticonvulsant activity (ED₅₀ = 14.5 mg/kg in mice) but undergoes extensive reductive metabolism .
- Fluorophenyl-substituted analogs may exhibit improved metabolic stability due to fluorine’s resistance to oxidative degradation, though this remains untested .
C. Fluorescent Probes
- 2-(4-(Fluorosulfonyloxy)phenyl)benzoxazole exhibits strong fluorescence, making it suitable for OLEDs and bioimaging .
- The fluorophenyl group in this compound could similarly enhance fluorescence quantum yield, but its rigid structure may limit solvatochromic effects compared to benzoxazoles .
Physicochemical and Pharmacokinetic Properties
- Lipophilicity: Fluorine increases logP compared to non-fluorinated analogs (e.g., 3-phenyl-2,1-benzisoxazole), improving blood-brain barrier penetration .
- Metabolism : Fluorine reduces oxidative metabolism, as seen in zonisamide’s sulfamoyl group, which undergoes glucuronidation rather than oxidation .
Data Tables
Key Findings and Implications
- Synthesis : Fluorophenyl substitution complicates cross-coupling reactions due to electronic deactivation but enhances thermal stability marginally compared to nitro-substituted analogs .
- Biological Activity : Fluorine’s electronegativity improves binding in MAO-B inhibitors and anticonvulsants but is less effective than nitriles or sulfonamides .
Preparation Methods
Cyclization of Nitroarenes with Arylacetonitriles
The most widely reported synthesis of 3-(4-fluorophenyl)-2,1-benzisoxazole involves the base-mediated cyclization of nitroarenes with arylacetonitriles. This method leverages the electron-deficient nature of nitroarenes to facilitate nucleophilic aromatic substitution, followed by intramolecular cyclization.
Reaction Mechanism and General Procedure
The reaction proceeds via a σ-adduct intermediate (Scheme 1). A nitroarene, such as 1-chloro-4-nitrobenzene, reacts with 4-fluorophenylacetonitrile in the presence of a strong base (e.g., t-BuOK) and a chlorotrialkylsilane in tetrahydrofuran (THF). The base deprotonates the arylacetonitrile, generating a nitrile-stabilized carbanion that attacks the nitroarene’s aromatic ring. Subsequent cyclization and elimination of HCl yield the benzisoxazole core .
Optimized Conditions :
-
Solvent : THF or ethanol
-
Base : t-BuOK (1.1 equiv)
-
Additive : Chlorotriethylsilane (1.2 equiv)
-
Temperature : Room temperature to 60°C
-
Time : 2–6 hours
Under these conditions, yields range from 65% to 82%, depending on the substituents’ electronic effects .
Substrate Scope and Limitations
The method is highly versatile for nitroarenes activated by electron-withdrawing groups (e.g., -NO₂, -CF₃). However, steric hindrance from ortho-substituents reduces reactivity. For example, 2-methyl-4-nitrochlorobenzene yields only 48% product under identical conditions .
One-Pot Oximation-Cyclization Strategy
A patent-pending one-pot method simplifies the synthesis by combining oximation and cyclization steps, eliminating intermediate isolation (Scheme 2) .
Procedure and Conditions
4-(2,4-Difluorobenzoyl)piperidine hydrochloride is dissolved in methanol, treated with hydroxylamine hydrochloride and potassium hydroxide, and heated at 40–45°C for 24 hours. Concentrated HCl is added to acidify the mixture, precipitating the product after cooling .
Key Advantages :
-
Solvent : Methanol (low cost, easy recovery)
-
Base : KOH (replaces triethylamine, reducing environmental toxicity)
-
Yield : 78–85%
-
Purity : >99% by HPLC
This method avoids hazardous reagents like triethylamine and streamlines purification, making it industrially viable .
Comparative Analysis of Synthetic Methods
The one-pot method excels in cost and scalability, whereas nitroarene cyclization offers broader substrate flexibility. Bromination remains niche for specialized applications.
Industrial and Environmental Considerations
The replacement of triethylamine with KOH in the one-pot method reduces wastewater contamination . Additionally, methanol’s low toxicity and high recyclability align with green chemistry principles. In contrast, nitroarene cyclization generates stoichiometric HCl, necessitating neutralization steps .
Properties
Molecular Formula |
C13H8FNO |
---|---|
Molecular Weight |
213.21 g/mol |
IUPAC Name |
3-(4-fluorophenyl)-2,1-benzoxazole |
InChI |
InChI=1S/C13H8FNO/c14-10-7-5-9(6-8-10)13-11-3-1-2-4-12(11)15-16-13/h1-8H |
InChI Key |
JDHHXWXEFCKCJA-UHFFFAOYSA-N |
Canonical SMILES |
C1=CC2=C(ON=C2C=C1)C3=CC=C(C=C3)F |
Origin of Product |
United States |
Synthesis routes and methods
Procedure details
Disclaimer and Information on In-Vitro Research Products
Please be aware that all articles and product information presented on BenchChem are intended solely for informational purposes. The products available for purchase on BenchChem are specifically designed for in-vitro studies, which are conducted outside of living organisms. In-vitro studies, derived from the Latin term "in glass," involve experiments performed in controlled laboratory settings using cells or tissues. It is important to note that these products are not categorized as medicines or drugs, and they have not received approval from the FDA for the prevention, treatment, or cure of any medical condition, ailment, or disease. We must emphasize that any form of bodily introduction of these products into humans or animals is strictly prohibited by law. It is essential to adhere to these guidelines to ensure compliance with legal and ethical standards in research and experimentation.