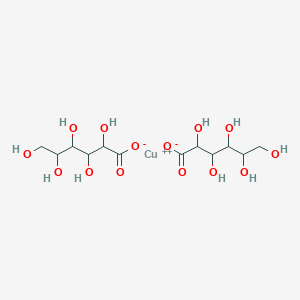
Copper D-gluconate
Overview
Description
Copper D-gluconate is the copper salt of D-gluconic acid. It appears as an odorless light blue or blue-green crystal or powder, which is easily soluble in water but insoluble in ethanol . This compound is commonly used as a dietary supplement to treat copper deficiency and as an ingredient in various products, including breath mints and fertilizers .
Mechanism of Action
Target of Action
Copper D-gluconate is a copper salt of D-gluconic acid . Copper is an essential micronutrient for both humans and animals, involved in various biological processes, including antioxidant defense, neuropeptide synthesis, and immune function . Copper is absorbed from the gut via high-affinity copper uptake protein and likely through low-affinity copper uptake protein and natural resistance-associated macrophage protein-2 .
Mode of Action
This compound interacts with its targets by binding to sulfur-containing biomolecules having a thiol or thioether group in the reduced state, whereas in the oxidized state, Cu2+ reacts primarily with oxygen or imidazole nitrogen groups . The interchange between Cu+ and Cu2+ may result in the generation of toxic reactive oxygen species (ROS) and other hydroxyl radicals .
Biochemical Pathways
Excessive copper exposure can modulate a large number of cytokines in both directions, increase and/or decrease through a variety of molecular and cellular signaling pathways including nuclear factor kappa-B (NF-κB) pathway, mitogen-activated protein kinase (MAPKs) pathway, JAK-STAT (Janus Kinase- signal transducer and activator of transcription) pathway, and NOD-like receptor protein 3 (NLRP3) inflammasome .
Pharmacokinetics (ADME Properties)
It’s known that copper is absorbed from the gut and transported to the liver . More research is needed to fully understand the ADME properties of this compound.
Result of Action
The molecular and cellular effects of this compound action are complex. Excessive copper exposure can cause damage to organs and cells, induce oxidative damage, inflammation, apoptosis, cell cycle arrest, and autophagy . It can also lead to protein aggregation, proteotoxic stress, and eventual cell death .
Action Environment
The action of this compound can be influenced by environmental factors. Copper concentration in agricultural soils depends on the concentration of parent materials, climate conditions, pedological factors, and addition through human activities . The design influences that affect the efficacy of this compound, and the possible environmental risks have also been briefly described .
Biochemical Analysis
Biochemical Properties
Copper D-gluconate plays a significant role in biochemical reactions. It interacts with various enzymes, proteins, and other biomolecules. For instance, it has been found to mitigate SARS-CoV-2 infection in Vero E6 cells . The study showed that cells treated with copper gluconate had a reduced infection rate compared to untreated cells .
Cellular Effects
This compound has notable effects on various types of cells and cellular processes. For example, it has been found to influence cell function by mitigating SARS-CoV-2 infection in Vero E6 cells . This suggests that copper gluconate could potentially influence cell signaling pathways, gene expression, and cellular metabolism.
Temporal Effects in Laboratory Settings
In laboratory settings, the effects of this compound can change over time. For instance, a study found that Vero E6 cells treated with this compound showed a reduced infection rate of SARS-CoV-2 over a 48-hour period . This suggests that this compound may have long-term effects on cellular function in in vitro studies.
Preparation Methods
Synthetic Routes and Reaction Conditions: Copper D-gluconate is typically synthesized by reacting gluconic acid solutions with cupric oxide or basic cupric carbonate . The reaction involves the following steps:
Preparation of Gluconic Acid: Gluconic acid is produced by the fermentation and oxidation of glucose using black mold.
Reaction with Copper Source: The gluconic acid solution is then reacted with cupric oxide or basic cupric carbonate to form this compound.
Industrial Production Methods: In industrial settings, the production of this compound involves the addition of basic copper carbonate to a gluconolactone solution, followed by stirring and crystallization . This method is favored for its simplicity, low energy consumption, and high yield .
Chemical Reactions Analysis
Types of Reactions: Copper D-gluconate undergoes various chemical reactions, including:
Oxidation: this compound can participate in oxidation reactions due to the presence of copper ions.
Reduction: The compound can also undergo reduction reactions, where copper ions are reduced to their elemental form.
Substitution: this compound can participate in substitution reactions, where the copper ion is replaced by another metal ion.
Common Reagents and Conditions:
Oxidizing Agents: Common oxidizing agents include hydrogen peroxide and potassium permanganate.
Reducing Agents: Common reducing agents include sodium borohydride and hydrazine.
Substitution Reagents: Substitution reactions often involve other metal salts or complexing agents.
Major Products: The major products formed from these reactions depend on the specific reagents and conditions used. For example, oxidation reactions may produce copper oxides, while reduction reactions may yield elemental copper .
Scientific Research Applications
Copper D-gluconate has a wide range of scientific research applications, including:
Comparison with Similar Compounds
- Zinc D-gluconate
- Iron D-gluconate
- Magnesium D-gluconate
- Calcium D-gluconate
Properties
IUPAC Name |
copper;(2R,3S,4R,5R)-2,3,4,5,6-pentahydroxyhexanoate | |
---|---|---|
Source | PubChem | |
URL | https://pubchem.ncbi.nlm.nih.gov | |
Description | Data deposited in or computed by PubChem | |
InChI |
InChI=1S/2C6H12O7.Cu/c2*7-1-2(8)3(9)4(10)5(11)6(12)13;/h2*2-5,7-11H,1H2,(H,12,13);/q;;+2/p-2/t2*2-,3-,4+,5-;/m11./s1 | |
Source | PubChem | |
URL | https://pubchem.ncbi.nlm.nih.gov | |
Description | Data deposited in or computed by PubChem | |
InChI Key |
OCUCCJIRFHNWBP-IYEMJOQQSA-L | |
Source | PubChem | |
URL | https://pubchem.ncbi.nlm.nih.gov | |
Description | Data deposited in or computed by PubChem | |
Canonical SMILES |
C(C(C(C(C(C(=O)[O-])O)O)O)O)O.C(C(C(C(C(C(=O)[O-])O)O)O)O)O.[Cu+2] | |
Source | PubChem | |
URL | https://pubchem.ncbi.nlm.nih.gov | |
Description | Data deposited in or computed by PubChem | |
Isomeric SMILES |
C([C@H]([C@H]([C@@H]([C@H](C(=O)[O-])O)O)O)O)O.C([C@H]([C@H]([C@@H]([C@H](C(=O)[O-])O)O)O)O)O.[Cu+2] | |
Source | PubChem | |
URL | https://pubchem.ncbi.nlm.nih.gov | |
Description | Data deposited in or computed by PubChem | |
Molecular Formula |
C12H22CuO14 | |
Source | PubChem | |
URL | https://pubchem.ncbi.nlm.nih.gov | |
Description | Data deposited in or computed by PubChem | |
DSSTOX Substance ID |
DTXSID4035960 | |
Record name | Copper gluconate | |
Source | EPA DSSTox | |
URL | https://comptox.epa.gov/dashboard/DTXSID4035960 | |
Description | DSSTox provides a high quality public chemistry resource for supporting improved predictive toxicology. | |
Molecular Weight |
453.84 g/mol | |
Source | PubChem | |
URL | https://pubchem.ncbi.nlm.nih.gov | |
Description | Data deposited in or computed by PubChem | |
CAS No. |
527-09-3 | |
Record name | Bis(D-gluconato-κO1,κO2)copper | |
Source | CAS Common Chemistry | |
URL | https://commonchemistry.cas.org/detail?cas_rn=527-09-3 | |
Description | CAS Common Chemistry is an open community resource for accessing chemical information. Nearly 500,000 chemical substances from CAS REGISTRY cover areas of community interest, including common and frequently regulated chemicals, and those relevant to high school and undergraduate chemistry classes. This chemical information, curated by our expert scientists, is provided in alignment with our mission as a division of the American Chemical Society. | |
Explanation | The data from CAS Common Chemistry is provided under a CC-BY-NC 4.0 license, unless otherwise stated. | |
Record name | Copper gluconate [USP] | |
Source | ChemIDplus | |
URL | https://pubchem.ncbi.nlm.nih.gov/substance/?source=chemidplus&sourceid=0000527093 | |
Description | ChemIDplus is a free, web search system that provides access to the structure and nomenclature authority files used for the identification of chemical substances cited in National Library of Medicine (NLM) databases, including the TOXNET system. | |
Record name | Copper gluconate | |
Source | DrugBank | |
URL | https://www.drugbank.ca/drugs/DB11246 | |
Description | The DrugBank database is a unique bioinformatics and cheminformatics resource that combines detailed drug (i.e. chemical, pharmacological and pharmaceutical) data with comprehensive drug target (i.e. sequence, structure, and pathway) information. | |
Explanation | Creative Common's Attribution-NonCommercial 4.0 International License (http://creativecommons.org/licenses/by-nc/4.0/legalcode) | |
Record name | Copper gluconate | |
Source | EPA DSSTox | |
URL | https://comptox.epa.gov/dashboard/DTXSID4035960 | |
Description | DSSTox provides a high quality public chemistry resource for supporting improved predictive toxicology. | |
Record name | COPPER GLUCONATE | |
Source | FDA Global Substance Registration System (GSRS) | |
URL | https://gsrs.ncats.nih.gov/ginas/app/beta/substances/RV823G6G67 | |
Description | The FDA Global Substance Registration System (GSRS) enables the efficient and accurate exchange of information on what substances are in regulated products. Instead of relying on names, which vary across regulatory domains, countries, and regions, the GSRS knowledge base makes it possible for substances to be defined by standardized, scientific descriptions. | |
Explanation | Unless otherwise noted, the contents of the FDA website (www.fda.gov), both text and graphics, are not copyrighted. They are in the public domain and may be republished, reprinted and otherwise used freely by anyone without the need to obtain permission from FDA. Credit to the U.S. Food and Drug Administration as the source is appreciated but not required. | |
Retrosynthesis Analysis
AI-Powered Synthesis Planning: Our tool employs the Template_relevance Pistachio, Template_relevance Bkms_metabolic, Template_relevance Pistachio_ringbreaker, Template_relevance Reaxys, Template_relevance Reaxys_biocatalysis model, leveraging a vast database of chemical reactions to predict feasible synthetic routes.
One-Step Synthesis Focus: Specifically designed for one-step synthesis, it provides concise and direct routes for your target compounds, streamlining the synthesis process.
Accurate Predictions: Utilizing the extensive PISTACHIO, BKMS_METABOLIC, PISTACHIO_RINGBREAKER, REAXYS, REAXYS_BIOCATALYSIS database, our tool offers high-accuracy predictions, reflecting the latest in chemical research and data.
Strategy Settings
Precursor scoring | Relevance Heuristic |
---|---|
Min. plausibility | 0.01 |
Model | Template_relevance |
Template Set | Pistachio/Bkms_metabolic/Pistachio_ringbreaker/Reaxys/Reaxys_biocatalysis |
Top-N result to add to graph | 6 |
Feasible Synthetic Routes
Q1: How does Copper D-gluconate affect the expression of β3 integrin subunit in vitro?
A1: In the study using Ishikawa cells (a human endometrial adenocarcinoma cell line), this compound was found to upregulate the expression of the β3 integrin subunit. [] This effect was observed both in the presence and absence of epidermal growth factor (EGF), with the most significant upregulation seen when both this compound and EGF were present. [] This finding is interesting because it contrasts with in vivo observations from Copper IUD users, where a reduction in β3 integrin subunit expression during the implantation window is seen. []
Q2: What are the possible reasons for the discrepancy between in vitro and in vivo findings regarding the effect of copper ions on β3 integrin subunit expression?
A2: The researchers propose that the differences between the in vitro study using this compound and in vivo observations in Copper IUD users might be attributed to other factors present in the in vivo environment. [] One key factor could be the inflammatory response elicited by the presence of IUDs in the uterus. [] This inflammatory milieu might influence β3 integrin subunit expression differently compared to the controlled environment of in vitro cell culture. Further research is needed to elucidate the interplay between copper ions, inflammation, and β3 integrin subunit expression in vivo.
Disclaimer and Information on In-Vitro Research Products
Please be aware that all articles and product information presented on BenchChem are intended solely for informational purposes. The products available for purchase on BenchChem are specifically designed for in-vitro studies, which are conducted outside of living organisms. In-vitro studies, derived from the Latin term "in glass," involve experiments performed in controlled laboratory settings using cells or tissues. It is important to note that these products are not categorized as medicines or drugs, and they have not received approval from the FDA for the prevention, treatment, or cure of any medical condition, ailment, or disease. We must emphasize that any form of bodily introduction of these products into humans or animals is strictly prohibited by law. It is essential to adhere to these guidelines to ensure compliance with legal and ethical standards in research and experimentation.