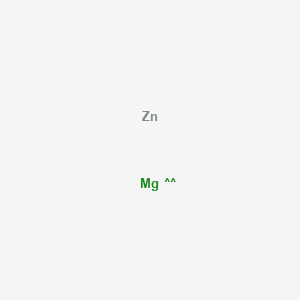
Magnesium-ZINC
- Click on QUICK INQUIRY to receive a quote from our team of experts.
- With the quality product at a COMPETITIVE price, you can focus more on your research.
Description
Magnesium-zinc (Mg-Zn) compounds encompass a range of materials, including alloys, oxides, and coordination complexes, which exhibit unique physicochemical properties. Mg-Zn alloys, for instance, are lightweight (density ~1.8–2.0 g/cm³) and biodegradable, making them promising for biomedical implants like stents and screws . Their microstructure and mechanical properties can be tailored by varying Zn content (typically 1–6 wt%) or incorporating nanoparticle reinforcements (e.g., Al₂O₃, TiC), enhancing tensile strength (up to 250 MPa) and ductility . Thermodynamically, Mg-Zn systems form intermetallic phases such as MgZn₂ and Mg₂Zn₃, with phase diagrams well-characterized up to 50 at% Zn . In natural settings, Zn²⁺ can substitute Mg²⁺ in minerals due to their similar ionic radii (~0.74 Å for Mg²⁺ vs. 0.75 Å for Zn²⁺), as observed in automolite (Zn-spinel) and franklinite .
Q & A
Basic Research Questions
Q. What methodologies are recommended for assessing zinc deficiency in experimental studies, and how can confounding variables be minimized?
- Answer : Zinc deficiency studies should employ standardized protocols, such as those outlined in meta-analyses using the AHRQ cross-sectional study checklist . Key steps include:
- Data Extraction : Collect zinc levels, deficiency rates, and covariates (e.g., dietary intake, geographic location).
- Quality Scoring : Use an 11-item checklist to classify studies as low/medium/high quality, prioritizing high-quality datasets.
- Statistical Adjustment : Apply multivariate regression to account for confounders like age, sex, and environmental zinc availability.
- Validation : Cross-reference findings with stable isotope-based studies to resolve discrepancies in factorial estimates .
Q. How can zinc speciation in environmental or biological systems be analytically determined?
- Answer : Speciation analysis requires a combination of:
- Modeling : Calculate equilibrium distributions of Zn(II) complexes (e.g., with chloride, humic acids) using pH-dependent solubility models .
- Experimental Techniques : Use ICP-MS for total zinc quantification and EXAFS/XANES for ligand-specific binding analysis.
- Bioavailability Assessment : Measure organically bound fractions (e.g., via sequential extraction in soils) and correlate with desorption rates at varying pH levels .
Q. What synthesis methods are effective for producing Mg-Zn alloys with controlled microstructures?
- Answer : Key approaches include:
- Hot Deformation : Optimize temperature (250–400°C) and strain rate to refine grain structures in Mg-Zn alloys, as demonstrated in texture evolution studies .
- Micro-Alloying : Add elements like neodymium to suppress recrystallization and enhance mechanical properties .
- Electrodeposition : Apply coatings (e.g., dicalcium phosphate dihydrate) to improve corrosion resistance and biocompatibility .
Advanced Research Questions
Q. How do microstructural changes in Mg-Zn alloys during hot deformation influence mechanical properties, and what experimental designs capture these dynamics?
- Answer :
- Experimental Design : Use in-situ EBSD (electron backscatter diffraction) to track grain boundary migration and dislocation density changes under varying strain rates .
- Data Interpretation : Correlate texture evolution (e.g., basal vs. non-basal slip systems) with tensile/compressive strength measurements.
- Contradictions : Resolve discrepancies in recrystallization kinetics by comparing alloys with/without micro-alloying elements (e.g., Nd) .
Q. What strategies address contradictions in zinc bioavailability data across different experimental models?
- Answer :
- Source Analysis : Identify whether discrepancies arise from methodological differences (e.g., in vitro vs. in vivo assays) or environmental variables (e.g., soil organic content) .
- Unified Frameworks : Adopt tiered testing protocols, starting with computational speciation models and validating via isotopic tracer studies in target populations .
- Principal Contradiction Analysis : Determine if bioavailability is primarily limited by solubility (pH-dependent) or ligand competition (e.g., Fe/Ca interactions) .
Q. How can researchers optimize Mg-Zn alloy coatings for biomedical applications while balancing degradation rates and biocompatibility?
- Answer :
- Coating Fabrication : Use pulsed electrodeposition to create uniform DCPD (dicalcium phosphate dihydrate) layers, characterized via XRD/SEM for crystallinity and adhesion .
- In Vitro Testing : Immerse coated alloys in simulated body fluid (SBF) to measure degradation kinetics (mass loss) and ion release profiles (ICP-OES).
- Contradiction Resolution : Address conflicting reports on corrosion resistance by standardizing testing conditions (e.g., pH 7.4, 37°C) .
Q. Key Recommendations for Researchers
Comparison with Similar Compounds
Mechanical Properties
Mg-Zn alloys are compared with other biodegradable metals (e.g., Mg-Al, Zn-Cu) in Table 1.
Table 1: Mechanical Properties of Mg-Zn Alloys vs. Similar Materials
Property | Mg-Zn (4% Zn) | Mg-Al (3% Al) | Zn-Cu (1% Cu) | Reference |
---|---|---|---|---|
Tensile Strength (MPa) | 220–250 | 180–200 | 150–170 | |
Corrosion Rate (mm/year) | 0.5–1.2 | 1.5–2.5 | 0.3–0.8 | |
Ductility (% elongation) | 10–15 | 5–8 | 2–5 |
Key Findings:
- Mg-Zn alloys outperform Mg-Al in strength and ductility due to refined grain structures and reduced hydrogen embrittlement .
- Zn-Cu alloys exhibit slower corrosion but lower mechanical strength, limiting their use in load-bearing applications .
Table 2: Biocompatibility Comparison
Parameter | Mg-Zn Alloy | Pure Mg | Stainless Steel | Reference |
---|---|---|---|---|
Hydrogen Gas Release | Moderate | High | None | |
Daily Tolerance (mg/day) | 10–15 (Zn) | 300–400 (Mg) | N/A | |
Degradation Rate | Adjustable | Rapid | Negligible |
Key Findings:
- Mg-Zn alloys mitigate hydrogen gas release compared to pure Mg, but Zn’s lower daily tolerance necessitates controlled degradation .
- Stainless steel, while inert, lacks biodegradability, requiring secondary removal surgeries .
Chemical Stability and Reactivity
- Oxides: MgO and ZnO nanoparticles differ in reactivity. ZnO exhibits superior photocatalytic activity but higher cytotoxicity compared to MgO .
- Ferrites : Mg-Zn ferrites (e.g., Mg₀.₅Zn₀.₅Fe₂O₄) show enhanced gas sensing performance (e.g., LPG detection) compared to pure Mg or Zn ferrites due to tailored bandgap energies .
Thermal and Electrical Properties
- Thermal Conductivity : Mg-Zn alloys (~120 W/m·K) are less conductive than Al alloys (~200 W/m·K) but superior to polymers (<1 W/m·K) .
- Electrical Resistivity : Mg-Zn ferrites exhibit resistivity ~10⁶ Ω·cm, suitable for high-frequency applications, whereas Cu-Zn ferrites are more conductive (~10³ Ω·cm) .
Occurrence and Substitution in Natural Compounds
Zn²⁺ substitutes Mg²⁺ in magnesian minerals (e.g., spinels) due to isomorphic replacement, though Zn-rich minerals (e.g., franklinite with 17% ZnO) are rarer than Mg-dominant variants . This substitution is less common than Fe²⁺/Mn²⁺ replacement in Mg minerals, reflecting Zn’s lower crustal abundance .
Properties
CAS No. |
12032-46-1 |
---|---|
Molecular Formula |
MgZn |
Molecular Weight |
89.7 g/mol |
IUPAC Name |
magnesium;zinc |
InChI |
InChI=1S/Mg.Zn |
InChI Key |
PGTXKIZLOWULDJ-UHFFFAOYSA-N |
Canonical SMILES |
[Mg].[Zn] |
Origin of Product |
United States |
Disclaimer and Information on In-Vitro Research Products
Please be aware that all articles and product information presented on BenchChem are intended solely for informational purposes. The products available for purchase on BenchChem are specifically designed for in-vitro studies, which are conducted outside of living organisms. In-vitro studies, derived from the Latin term "in glass," involve experiments performed in controlled laboratory settings using cells or tissues. It is important to note that these products are not categorized as medicines or drugs, and they have not received approval from the FDA for the prevention, treatment, or cure of any medical condition, ailment, or disease. We must emphasize that any form of bodily introduction of these products into humans or animals is strictly prohibited by law. It is essential to adhere to these guidelines to ensure compliance with legal and ethical standards in research and experimentation.