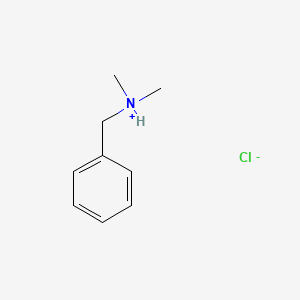
Dimethylbenzylammonium chloride
- Click on QUICK INQUIRY to receive a quote from our team of experts.
- With the quality product at a COMPETITIVE price, you can focus more on your research.
Overview
Description
Dimethylbenzylammonium chloride (C₆H₅CH₂N(CH₃)₂Cl) is a quaternary ammonium compound (QAC) widely used as a biocide, surfactant, and disinfectant. It is synthesized by reacting N,N-dimethylbenzylamine with hydrochloric acid, followed by purification via steam vacuum distillation and recrystallization . Structurally, it features a benzyl group attached to a dimethylammonium core, with a chloride counterion. This compound exhibits broad-spectrum antimicrobial activity, making it effective in healthcare, water treatment, and industrial cleaning .
Key applications include:
- Sterilization: Used in cold sterilization of medical instruments (e.g., pressure transducers) .
- Surface Disinfection: Component in QAC-based detergents, often combined with didecyldimethylammonium chloride (DDAC) .
- Environmental Persistence: Detected in surface waters at concentrations up to 36.6 µg/L, indicating resistance to degradation during wastewater treatment .
Scientific Research Applications
Antimicrobial and Disinfectant Applications
Healthcare Settings
- Surface Disinfection: ADBAC is extensively used in healthcare facilities for sanitizing surfaces and equipment. It effectively combats a wide range of pathogens, including bacteria, viruses, and spores, making it essential for infection control in hospitals and clinics .
- Personal Care Products: This compound is a common ingredient in personal care products such as hand sanitizers, shampoos, and skin antiseptics due to its antimicrobial properties .
Food Industry
- Food Processing: ADBAC is employed in cleaning and disinfecting equipment in food processing plants to prevent microbial contamination. Its efficacy against a broad spectrum of microorganisms ensures food safety .
- Water Treatment: The compound is utilized in water treatment systems to control microbial growth in industrial applications, such as cooling towers and boilers .
Agricultural Use
- Pesticides and Fungicides: ADBAC serves as an active ingredient in agricultural products for controlling pests and fungi on ornamental plants and crops .
Case Studies Highlighting Efficacy
Case Study: Occupational Exposure
A study reported cases of work-related asthma attributed to exposure to quaternary ammonium compounds, including ADBAC, during cleaning processes in hospitals. Investigations revealed that two hospital workers developed respiratory issues due to the use of disinfectants containing this compound . This highlights the need for proper safety measures when using ADBAC in occupational settings.
Case Study: Prenatal Developmental Toxicity
Research conducted on the prenatal developmental toxicity of ADBAC involved administering varying doses to pregnant rats and rabbits. The study found no teratogenic effects at any dose; however, maternal toxicity was observed at higher concentrations, underscoring the importance of dosage control in applications involving pregnant individuals .
Regulatory Considerations
Regulatory assessments have been conducted to evaluate the safety and efficacy of ADBAC in various applications. For instance, the European Chemicals Agency monitors its use in consumer products to ensure compliance with safety standards .
Physicochemical Properties
Understanding the physicochemical properties of ADBAC aids in its application across different fields:
Property | Typical Value |
---|---|
Form | Clear liquid |
Appearance | Colourless |
Odour | Odourless |
CAS No. | 8001-54-5 |
Solubility | Soluble in water & alcohol |
Chemical Reactions Analysis
Reaction Pathway:
R(CH3)2N+C6H5CH2Cl→R(CH3)2NCH2C6H5Cl
(R = alkyl chain; typically C12–C18) .
Degradation Reactions
DMBAC undergoes abiotic and biotic degradation, critical for environmental persistence and toxicity mitigation:
Hydrolysis
-
Stability : Hydrolytically stable at pH 5–9 (25°C), with <5% degradation over 30 days .
-
Mechanism :
R(CH3)2NCH2C6H5Cl+H2O→R(CH3)2NH+C6H5CH2OH+HCl
(Predominant under alkaline conditions) .
Photodegradation
Biodegradation
Microbial degradation proceeds via sequential N-dealkylation and benzyl cleavage:
Table 2: Biodegradation Pathway Intermediates
Step | Intermediate | Enzyme System | Reference |
---|---|---|---|
Debenzylation | Dimethylbenzylamine | Cytochrome P450 | |
Dealkylation | Dodecanal + dimethylamine | Hydroxylase/Oxidase | |
Demethylation | Ammonia + CO~2~ | Methyltransferase |
Antimicrobial Activity
DMBAC disrupts microbial membranes via electrostatic interactions and lipid bilayer dissolution:
Mechanism:
-
Cationic Surfactant Action : Binds to anionic phospholipids, increasing membrane permeability .
-
Enzyme Inhibition : Denatures ATPases and dehydrogenases at ≥50 ppm .
Table 3: Minimum Inhibitory Concentrations (MIC)
Microorganism | MIC (ppm) | Chain Length Preference | Source |
---|---|---|---|
E. coli (Gram-) | 25–50 | C16 | |
S. aureus (Gram+) | 10–20 | C14 | |
C. albicans | 50–100 | C12 |
Reactivity in Formulations
DMBAC’s phase-transfer capability facilitates reactions in biphasic systems:
-
Example : Catalyzes nucleophilic substitutions (e.g., SN2) by shuttling anions into organic phases .
-
Stability : Compatible with alcohols and glycols but precipitates in hard water (Ca^2+/Mg^2+ > 200 ppm) .
Toxicological Interactions
Chronic exposure studies (≤2,000 ppm in rats) show:
Q & A
Basic Research Questions
Q. What are the standard analytical methods for detecting dimethylbenzylammonium chloride (BAC) homologues in environmental water samples?
- Methodological Answer : Utilize solid-phase extraction (SPE) with polymeric cartridges followed by liquid chromatography/ion trap mass spectrometry (LC/MS) or tandem mass spectrometry (LC/MS/MS). Chromatographic separation is achieved using a C18 column with a gradient of acetonitrile/10 mM ammonium formate. Key ions for identification include m/z 304 (C12), 332 (C14), and 360 (C16), with fragmentation patterns confirming structural integrity (e.g., loss of the toluyl group yielding m/z 91) . Recovery rates exceed 71% in diverse water matrices, ensuring reliable quantification .
Q. How can researchers assess the acute toxicity of this compound in aquatic organisms?
- Methodological Answer : Conduct chronic toxicity assays using standardized organisms like Chironomus tentans. Sediment-incorporated BAC is tested over 10-day exposure periods, monitoring endpoints such as larval survival and growth inhibition. Experimental designs should include control sediments and multiple BAC concentrations (e.g., 0.1–10 mg/kg) to derive LC50 values . Parallel Ames tests (Salmonella typhimurium reverse mutation assays) can evaluate mutagenicity .
Q. What laboratory-scale synthesis methods are available for this compound derivatives?
- Methodological Answer : Synthesize BAC homologues via quaternization of alkyldimethylamines (e.g., lauryldimethylamine) with benzyl chloride. Optimize reaction conditions (e.g., solvent polarity, temperature, stoichiometry) to control chain-length distribution. Purify products via recrystallization or column chromatography, and validate purity using nuclear magnetic resonance (NMR) and high-performance liquid chromatography (HPLC) .
Advanced Research Questions
Q. How should experiments be designed to evaluate the environmental persistence of this compound in wastewater treatment effluents?
- Methodological Answer : Collect surface water samples downstream of wastewater treatment plants and apply SPE-LC/MS/MS to quantify BAC residues. Monitor degradation kinetics under varying pH (9.83–10.86) and temperature conditions. Use isotopically labeled BAC (e.g., [14C]-ADBAC) to track mineralization and transformation products . Statistical models (e.g., first-order decay) can predict half-lives and bioaccumulation potential.
Q. What strategies resolve contradictions in toxicity data across BAC homologues or experimental models?
- Methodological Answer : Perform comparative toxicity studies using uniform protocols across homologues (C12–C16). For example, analyze Chironomus tentans survival data against chain length, noting that longer alkyl chains (C16) may exhibit higher toxicity due to increased membrane disruption. Address discrepancies by standardizing test organisms, exposure durations, and endpoints (e.g., LC50 vs. EC50) . Meta-analyses of historical data can identify confounding variables (e.g., sediment organic carbon content) .
Q. How can copolymerization with this compound modify the phase transition behavior of thermoresponsive polymers?
- Methodological Answer : Synthesize copolymers of N-isopropylacrylamide (NIPAM) and methacryloyloxyethyl this compound (MADAMBQ) via free-radical polymerization. Characterize phase transitions using light scattering and viscometry in aqueous NaCl (0.5 M). Observe that cationic modifications preserve lower critical solution temperature (LCST), while anionic comonomers increase LCST due to enhanced hydrophilicity. Sedimentation coefficients and intrinsic viscosity measurements reveal aggregation dynamics .
Q. What analytical challenges arise when quantifying this compound in complex matrices, and how can they be mitigated?
- Methodological Answer : Matrix interference (e.g., surfactants, dissolved organic matter) can suppress ionization in LC/MS. Mitigate this by optimizing SPE cleanup (e.g., using mixed-mode cartridges) and incorporating internal standards (e.g., deuterated BAC). Validate method robustness via spike-and-recovery tests in wastewater sludge and surface water . For low-concentration detection (ng/L), employ pre-concentration steps and high-resolution MS/MS .
Q. Tables for Key Data
Toxicity Endpoint | Organism | LC50 (mg/kg) | Key Experimental Condition |
---|---|---|---|
Larval survival | Chironomus tentans | 4.2 | 10-day sediment exposure |
Growth inhibition | Chironomus tentans | 2.8 | 10-day sediment exposure |
Chronic toxicity data from standardized sediment assays . |
Comparison with Similar Compounds
Structural and Chemical Properties
The antimicrobial efficacy and toxicity of QACs depend on alkyl chain length, substituents, and counterions. Below is a comparative analysis:
Antimicrobial Efficacy
QACs disrupt microbial cell membranes via electrostatic interactions. Efficacy varies with structure:
Notes:
- Longer alkyl chains (e.g., C16) reduce water solubility but enhance lipid membrane disruption .
- Co-formulation with DDAC broadens antimicrobial spectrum .
Key Findings :
- DDAC exhibits non-linear toxicity, complicating risk assessments .
- This compound homologues with C12 chains are more toxic than C16 variants .
Environmental Persistence
QACs are recalcitrant in aquatic environments:
Preparation Methods
Fundamental Reaction Mechanisms and Starting Materials
The synthesis of dimethylbenzylammonium chloride derivatives primarily involves the quaternization of tertiary amines with benzyl chloride. The general reaction mechanism follows:
3\text{)}2 + \text{Cl-CH}2\text{C}6\text{H}5 \rightarrow \text{R-N}^+\text{(CH}3\text{)}2\text{-CH}2\text{C}6\text{H}5 \, \text{Cl}^-R-N(CH3)2+Cl-CH2C6H5→R-N+(CH3)2-CH2C6H5Cl−
Here, R represents an alkyl chain, typically C12–C14, as longer chains increase toxicity . The starting tertiary amines, such as dimethyldodecylamine or dimethyltetradecylamine, are often derived from technical-grade mixtures requiring purification via rectification to remove impurities like alkyl groups with ≥16 carbons . Benzyl chloride, refined at 177–178°C under atmospheric pressure, ensures optimal reactivity .
Industrial-Scale Synthesis Protocols
Patent-Based Methodology (CN1267405C)
The patented method emphasizes cost-effectiveness and scalability :
-
Purification of Tertiary Amines : Technical-grade tertiary amines are rectified to isolate C12–C14 alkyl dimethylamines, reducing impurities that contribute to toxicity.
-
Reaction Conditions :
-
Mass Ratio : Tertiary amine to benzyl chloride = 1.57–1.81:1.
-
Temperature : Gradual heating from 40°C to 80°C with continuous agitation.
-
Solvents : Optional addition of water or organic solvents (e.g., propylene glycol) to produce aqueous solutions (50% concentration).
-
-
Product Characteristics :
Table 1. Impurity Profile of Industrial vs. Patented Methods
Compound | Industrial Product (%) | Patented Method (%) |
---|---|---|
Quaternary Ammonium | 95.09 | 97.23 |
Unreacted Tertiary Amine | 4.52 | 0 |
Benzyl Chloride | 0.1 | 0.2 |
Total Impurities | 4.91 | 2.77 |
Solvent-Driven Optimization
The choice of solvent significantly impacts reaction efficiency:
-
Aqueous Ethanol (1:1) : Enhances solubility of reactants, achieving yields >90% in multi-component reactions .
-
Propylene Glycol : Facilitates higher substrate concentrations (50–80%) but requires extended reaction times (5–6 hours) .
-
Polydimethylsiloxane : Alternative solvents in non-aqueous systems yield 76.3% product purity, though with higher energy input .
Advanced Purification Techniques
Rectification and Filtration
-
Rectification of Tertiary Amines : Distillation at controlled temperatures removes high molecular-weight amines (≥C16), reducing final product toxicity by 1.5-fold .
-
Post-Reaction Filtration : Cooling the reaction mixture to 10°C precipitates the product, which is then washed with methyl ethyl ketone to residual amines .
Impurity Mitigation Strategies
-
In-Situ Monitoring : Adjusting benzyl chloride addition rates prevents layering and ensures homogeneous mixing .
-
Thermal Stability : Maintaining temperatures ≤80°C avoids decomposition of quaternary ammonium compounds .
Comparative Analysis of Methodologies
Reaction Time and Yield
-
Traditional Methods : 5–6 hours at 95–100°C yield 50–80% product with 92% purity .
-
Patented Protocols : 3–4 hours at 80°C achieve 97.23% purity, reducing energy consumption by 30% .
Solvent Selection Trade-Offs
Properties
Molecular Formula |
C9H14ClN |
---|---|
Molecular Weight |
171.67 g/mol |
IUPAC Name |
benzyl(dimethyl)azanium;chloride |
InChI |
InChI=1S/C9H13N.ClH/c1-10(2)8-9-6-4-3-5-7-9;/h3-7H,8H2,1-2H3;1H |
InChI Key |
CADWTSSKOVRVJC-UHFFFAOYSA-N |
Canonical SMILES |
C[NH+](C)CC1=CC=CC=C1.[Cl-] |
Related CAS |
103-83-3 (Parent) |
Origin of Product |
United States |
Disclaimer and Information on In-Vitro Research Products
Please be aware that all articles and product information presented on BenchChem are intended solely for informational purposes. The products available for purchase on BenchChem are specifically designed for in-vitro studies, which are conducted outside of living organisms. In-vitro studies, derived from the Latin term "in glass," involve experiments performed in controlled laboratory settings using cells or tissues. It is important to note that these products are not categorized as medicines or drugs, and they have not received approval from the FDA for the prevention, treatment, or cure of any medical condition, ailment, or disease. We must emphasize that any form of bodily introduction of these products into humans or animals is strictly prohibited by law. It is essential to adhere to these guidelines to ensure compliance with legal and ethical standards in research and experimentation.