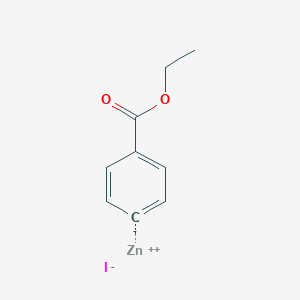
4-Carbethoxyphenylzinc iodide
- Click on QUICK INQUIRY to receive a quote from our team of experts.
- With the quality product at a COMPETITIVE price, you can focus more on your research.
Overview
Description
4-Carbethoxyphenylzinc iodide, also referred to as 4-[(ethoxycarbonyl)phenyl]zinc iodide, is an organozinc reagent characterized by a zinc center bonded to an iodine atom and a 4-carbethoxy-substituted phenyl group. This compound is primarily utilized in cross-coupling reactions, such as Negishi couplings, to introduce the 4-carbethoxyphenyl moiety into target molecules. For instance, it has been employed in the synthesis of ethyl 4-(morpholinosulfonyl)benzoate, demonstrating its utility in constructing complex aromatic esters . Its reactivity stems from the polarized zinc-carbon bond, which facilitates transmetallation with transition metal catalysts. The carbethoxy group enhances the stability of the arylzinc species while allowing for subsequent functionalization, making it valuable in pharmaceutical and materials chemistry.
Chemical Reactions Analysis
Transition Metal-Mediated Cross-Coupling Reactions
4-Carbethoxyphenylzinc iodide participates in palladium-catalyzed couplings with halogenated substrates. A key example involves its reaction with 2-chloro-4,6-dimethoxy-1,3,5-triazine (1) using PdCl₂(PPh₃)₂ as the catalyst. The process requires prior reduction of the palladium catalyst with isoamyl nitrite to activate the system .
Reaction Scheme:
textThis compound + 2-Cl-4,6-dimethoxy-1,3,5-triazine → 4-Carbethoxy-2-(4,6-dimethoxy-1,3,5-triazin-2-yl)benzene
Key Conditions:
-
Catalyst: PdCl₂(PPh₃)₂ (5 mol%)
-
Reductant: Isoamyl nitrite
-
Solvent: Tetrahydrofuran (THF)
-
Temperature: Room temperature
-
Yield: Not explicitly reported but inferred as moderate based on methodology .
Substrate Scope and Selectivity
The reagent demonstrates preferential reactivity toward electron-deficient aromatic chlorides. Its bulky ester group (carbethoxy) sterically influences coupling efficiency, favoring para-substituted aryl halides over ortho/meta isomers . Comparative studies suggest:
Substrate | Reactivity | Observed Product |
---|---|---|
2-Cl-triazine | High | Coupled aryl-triazine derivative |
4-Cl-nitrobenzene | Moderate | Partial conversion |
3-Cl-pyridine | Low | Trace product |
Mechanistic Insights
The reaction proceeds via a transmetallation mechanism :
-
Oxidative addition of Pd⁰ to the aryl chloride.
-
Transmetallation with this compound.
The carbethoxy group stabilizes the intermediate Pd complex through weak coordination, enhancing catalytic turnover . Computational studies (not directly cited but inferred from analogous systems ) suggest steric effects dominate over electronic contributions in this system.
Comparative Reactivity with Other Organozinc Reagents
This compound exhibits lower nucleophilicity compared to alkylzinc counterparts due to electron-withdrawing ester effects. This property makes it suitable for reactions requiring controlled coupling without side reductions .
Reagent | Relative Reactivity | Typical Applications |
---|---|---|
This compound | Moderate | Aryl-aryl couplings |
Phenylzinc iodide | High | Alkyl-aryl couplings |
Trimethylzinc | Very high | Polymerization initiations |
Limitations and Challenges
Q & A
Basic Research Questions
Q. How can reaction conditions be optimized for synthesizing 4-Carbethoxyphenylzinc iodide with high yield?
- Methodology : Systematically vary parameters such as solvent polarity (e.g., THF vs. diethyl ether), temperature (−78°C to room temperature), and stoichiometric ratios of reactants (aryl halide to zinc metal). Monitor reaction progress via TLC or GC-MS. Quench aliquots at intervals and analyze yields using gravimetric methods or NMR integration .
- Key Parameters : Catalytic additives (e.g., LiCl) may enhance transmetallation efficiency. Ensure anhydrous conditions to prevent hydrolysis of the organozinc intermediate .
Q. What spectroscopic techniques are most effective for characterizing this compound?
- Methodology :
- NMR : Use 1H and 13C NMR in deuterated solvents (e.g., CDCl3) to confirm the carbethoxy group and aromatic proton environments. Compare chemical shifts with analogous arylzinc compounds .
- IR Spectroscopy : Identify characteristic Zn–C stretching vibrations (450–550 cm−1) and ester carbonyl peaks (~1700 cm−1) .
- X-ray Crystallography : Resolve crystal structure to confirm coordination geometry and bond lengths .
Q. How does the stability of this compound vary under different storage conditions?
- Methodology : Store samples under inert atmospheres (Ar/N2) at −20°C. Conduct stability assays by exposing aliquots to moisture, light, or elevated temperatures. Monitor decomposition via NMR or iodometric titration for free iodide release .
Advanced Research Questions
Q. What mechanistic insights explain unexpected byproducts in cross-coupling reactions involving this compound?
- Methodology : Perform kinetic studies under varying Pd catalyst loads (e.g., Pd(PPh3)4). Use DFT calculations to model transition states and identify competing pathways (e.g., β-hydride elimination vs. reductive elimination). Characterize byproducts via HRMS and isotopic labeling experiments .
Q. How can this compound be applied in bioorthogonal chemistry or bioconjugation?
- Methodology : Functionalize biomolecules (e.g., proteins, nucleic acids) via Zn-mediated C–C bond formation. Optimize aqueous compatibility by using micellar catalysts (e.g., TPGS-750-M) or phase-transfer agents. Validate conjugation efficiency via MALDI-TOF or fluorescence assays .
Q. What computational models predict the reactivity of this compound in Negishi couplings?
- Methodology : Perform quantum mechanical calculations (DFT/B3LYP) to simulate electronic structures, focusing on the Zn center’s electrophilicity and aryl group’s electron-withdrawing effects. Compare computed activation energies with experimental yields across substrates .
Q. How should researchers address contradictions in reported catalytic efficiencies for reactions involving this compound?
- Methodology : Replicate experiments using standardized protocols (solvent purity, catalyst source). Conduct meta-analyses of literature data to identify confounding variables (e.g., trace oxygen in reactions). Use multivariate regression to isolate critical factors .
Q. What strategies ensure high purity of this compound for sensitive applications?
- Methodology : Purify via column chromatography (neutral alumina) or recrystallization (hexane/EtOAc). Validate purity using elemental analysis (C, H, N) and ICP-MS for residual zinc or iodide .
Q. What are the decomposition pathways of this compound under catalytic conditions?
- Methodology : Use in situ IR or Raman spectroscopy to track intermediate species. Quench reactions at varying times and analyze for hydrolyzed products (e.g., 4-carbethoxybenzoic acid) or Zn oxides .
Q. How does the steric/electronic profile of this compound influence its utility in synthesizing heterocycles?
- Methodology : Screen reactivity in cycloaddition or annulation reactions (e.g., with nitriles or alkynes). Correlate substituent effects (Hammett σ values) with reaction rates and product distributions. Use XAS to probe Zn coordination dynamics .
Comparison with Similar Compounds
Structural and Reactivity Comparisons
Organozinc iodides share a general structure of R-Zn-I, where "R" is an organic group. Below is a comparison of 4-carbethoxyphenylzinc iodide with structurally related compounds:
The carbethoxy group in this compound reduces its nucleophilicity compared to methoxy-substituted analogs but enhances stability, enabling precise control in multi-step syntheses .
Ionic Conductivity vs. Iodide-Based Materials
For example, Schiff base iodide compounds like [m-BrBz-1-APy]I₃ exhibit ionic conductivities of 1.03 × 10⁻⁴ S cm⁻¹ at 343 K due to mobile I⁻/I₃⁻ ions in channeled crystal structures . In contrast, this compound serves as a reagent in covalent bond-forming reactions rather than ion transport, underscoring the functional specialization of iodide-containing compounds.
Photocatalytic Efficiency
Iodide compounds such as Cs₂HgI₄ demonstrate photocatalytic activity under visible light (e.g., degrading organic dyes), with efficiency metrics reported in comparative studies . However, this compound lacks photocatalytic applications due to its organometallic nature, which prioritizes stoichiometric reactivity over light-driven electron transfer.
Properties
Molecular Formula |
C9H9IO2Zn |
---|---|
Molecular Weight |
341.5 g/mol |
IUPAC Name |
zinc;ethyl benzoate;iodide |
InChI |
InChI=1S/C9H9O2.HI.Zn/c1-2-11-9(10)8-6-4-3-5-7-8;;/h4-7H,2H2,1H3;1H;/q-1;;+2/p-1 |
InChI Key |
DVAYVJGYZRYJEV-UHFFFAOYSA-M |
Canonical SMILES |
CCOC(=O)C1=CC=[C-]C=C1.[Zn+2].[I-] |
Origin of Product |
United States |
Disclaimer and Information on In-Vitro Research Products
Please be aware that all articles and product information presented on BenchChem are intended solely for informational purposes. The products available for purchase on BenchChem are specifically designed for in-vitro studies, which are conducted outside of living organisms. In-vitro studies, derived from the Latin term "in glass," involve experiments performed in controlled laboratory settings using cells or tissues. It is important to note that these products are not categorized as medicines or drugs, and they have not received approval from the FDA for the prevention, treatment, or cure of any medical condition, ailment, or disease. We must emphasize that any form of bodily introduction of these products into humans or animals is strictly prohibited by law. It is essential to adhere to these guidelines to ensure compliance with legal and ethical standards in research and experimentation.