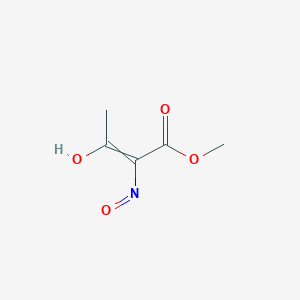
Methyl 2-(hydroxyimino)-3-oxobutanoate
- Click on QUICK INQUIRY to receive a quote from our team of experts.
- With the quality product at a COMPETITIVE price, you can focus more on your research.
Overview
Description
Methyl 2-(hydroxyimino)-3-oxobutanoate is an organic compound with the molecular formula C5H7NO4 It is a derivative of acetoacetic acid and contains both an ester and an oxime functional group
Preparation Methods
Synthetic Routes and Reaction Conditions
Methyl 2-(hydroxyimino)-3-oxobutanoate can be synthesized through the reaction of methyl acetoacetate with hydroxylamine hydrochloride in the presence of a base such as sodium acetate. The reaction typically occurs in an aqueous or alcoholic medium at elevated temperatures. The general reaction scheme is as follows:
CH3COCH2COOCH3+NH2OH⋅HCl→CH3C(NOH)CH2COOCH3+H2O+NaCl
Industrial Production Methods
Industrial production methods for methyl 2-hydroxyiminoacetoacetate are similar to laboratory synthesis but are scaled up to accommodate larger quantities. The process involves continuous flow reactors to maintain consistent reaction conditions and optimize yield. The use of catalysts and automated systems ensures efficiency and cost-effectiveness.
Chemical Reactions Analysis
Types of Reactions
Methyl 2-(hydroxyimino)-3-oxobutanoate undergoes various chemical reactions, including:
Oxidation: The oxime group can be oxidized to form nitrile oxides.
Reduction: The oxime group can be reduced to form amines.
Substitution: The ester group can undergo nucleophilic substitution reactions.
Common Reagents and Conditions
Oxidation: Reagents such as hydrogen peroxide or peracids are commonly used.
Reduction: Reagents like sodium borohydride or lithium aluminum hydride are employed.
Substitution: Nucleophiles such as amines or alcohols can react with the ester group under basic or acidic conditions.
Major Products Formed
Oxidation: Nitrile oxides.
Reduction: Amines.
Substitution: Substituted esters or amides.
Scientific Research Applications
Methyl 2-(hydroxyimino)-3-oxobutanoate has several applications in scientific research:
Chemistry: Used as a building block in organic synthesis for the preparation of heterocyclic compounds.
Biology: Investigated for its potential as an enzyme inhibitor.
Medicine: Explored for its potential therapeutic properties, including antimicrobial and anticancer activities.
Industry: Utilized in the synthesis of fine chemicals and pharmaceuticals.
Mechanism of Action
The mechanism of action of methyl 2-hydroxyiminoacetoacetate involves its interaction with various molecular targets. The oxime group can form hydrogen bonds with active sites of enzymes, potentially inhibiting their activity. The ester group can undergo hydrolysis, releasing active metabolites that exert biological effects.
Comparison with Similar Compounds
Similar Compounds
Methyl acetoacetate: Lacks the oxime group, making it less reactive in certain reactions.
Ethyl 2-hydroxyiminoacetoacetate: Similar structure but with an ethyl ester group instead of a methyl ester group.
2-Hydroxyiminoacetoacetic acid: The free acid form, which is more acidic and less stable than the ester derivative.
Uniqueness
Methyl 2-(hydroxyimino)-3-oxobutanoate is unique due to the presence of both ester and oxime functional groups, which confer distinct reactivity and versatility in synthetic applications. Its ability to undergo a wide range of chemical reactions makes it a valuable compound in organic synthesis and research.
Properties
IUPAC Name |
methyl 3-hydroxy-2-nitrosobut-2-enoate |
Source
|
---|---|---|
Details | Computed by LexiChem 2.6.6 (PubChem release 2019.06.18) | |
Source | PubChem | |
URL | https://pubchem.ncbi.nlm.nih.gov | |
Description | Data deposited in or computed by PubChem | |
InChI |
InChI=1S/C5H7NO4/c1-3(7)4(6-9)5(8)10-2/h7H,1-2H3 |
Source
|
Details | Computed by InChI 1.0.5 (PubChem release 2019.06.18) | |
Source | PubChem | |
URL | https://pubchem.ncbi.nlm.nih.gov | |
Description | Data deposited in or computed by PubChem | |
InChI Key |
HBNXCPZHXHOZBN-UHFFFAOYSA-N |
Source
|
Details | Computed by InChI 1.0.5 (PubChem release 2019.06.18) | |
Source | PubChem | |
URL | https://pubchem.ncbi.nlm.nih.gov | |
Description | Data deposited in or computed by PubChem | |
Canonical SMILES |
CC(=C(C(=O)OC)N=O)O |
Source
|
Details | Computed by OEChem 2.1.5 (PubChem release 2019.06.18) | |
Source | PubChem | |
URL | https://pubchem.ncbi.nlm.nih.gov | |
Description | Data deposited in or computed by PubChem | |
Molecular Formula |
C5H7NO4 |
Source
|
Details | Computed by PubChem 2.1 (PubChem release 2019.06.18) | |
Source | PubChem | |
URL | https://pubchem.ncbi.nlm.nih.gov | |
Description | Data deposited in or computed by PubChem | |
Molecular Weight |
145.11 g/mol |
Source
|
Details | Computed by PubChem 2.1 (PubChem release 2021.05.07) | |
Source | PubChem | |
URL | https://pubchem.ncbi.nlm.nih.gov | |
Description | Data deposited in or computed by PubChem | |
Disclaimer and Information on In-Vitro Research Products
Please be aware that all articles and product information presented on BenchChem are intended solely for informational purposes. The products available for purchase on BenchChem are specifically designed for in-vitro studies, which are conducted outside of living organisms. In-vitro studies, derived from the Latin term "in glass," involve experiments performed in controlled laboratory settings using cells or tissues. It is important to note that these products are not categorized as medicines or drugs, and they have not received approval from the FDA for the prevention, treatment, or cure of any medical condition, ailment, or disease. We must emphasize that any form of bodily introduction of these products into humans or animals is strictly prohibited by law. It is essential to adhere to these guidelines to ensure compliance with legal and ethical standards in research and experimentation.