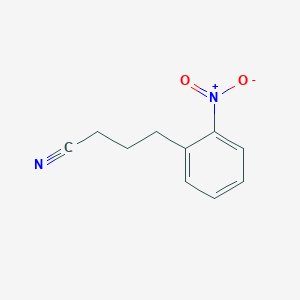
4-(2-Nitrophenyl)butyronitrile
Overview
Description
4-(2-Nitrophenyl)butyronitrile is an organic compound featuring a nitrile group (-CN) attached to a four-carbon chain, which is further substituted with a 2-nitrophenyl ring. This compound is primarily used as a synthetic intermediate in the production of 1,5-naphthalenediamine, a precursor for dyes and pharmaceuticals. Its synthesis involves the reaction of ortho-nitrotoluene with acrylonitrile, followed by reduction and cyclization steps . The ortho-substituted nitro group plays a critical role in directing reactivity during cyclization, enabling the formation of dihydro-naphthylamine derivatives .
Scientific Research Applications
Chemical Synthesis
4-(2-Nitrophenyl)butyronitrile is primarily synthesized through the reaction of ortho-nitrotoluene and acrylonitrile. This process typically occurs under basic conditions, utilizing catalysts such as sodium hydroxide or potassium hydroxide. The reaction can be optimized at temperatures ranging from 20 °C to 75 °C, with a focus on achieving high yields and purity of the final product .
Synthesis Process Overview
Step | Description |
---|---|
1 | React ortho-nitrotoluene with acrylonitrile in the presence of a base. |
2 | Maintain reaction temperatures between 20 °C and 75 °C for optimal yield. |
3 | Isolate and purify the resulting this compound. |
Biological Activities
Research indicates that this compound exhibits various biological activities that make it a candidate for further investigation in medicinal chemistry.
- Antimicrobial Properties : Studies have suggested that compounds containing nitrophenyl groups can possess antimicrobial activity, potentially making this compound useful in developing new antibiotics or antiseptics .
- Anti-inflammatory Effects : Preliminary data indicate that derivatives of butyronitriles may exhibit anti-inflammatory properties, which could be beneficial in treating inflammatory diseases .
- Potential as a Drug Intermediate : The compound serves as an intermediate in synthesizing more complex molecules that may have therapeutic effects against various diseases .
Case Study 1: Synthesis of Biologically Active Compounds
A notable study involved the synthesis of various biaryl substituted compounds using this compound as a precursor. The resulting compounds demonstrated enhanced biological activities, showcasing the utility of this nitrile in drug development .
Case Study 2: Development of Antimicrobial Agents
In another research project, scientists explored the antimicrobial properties of nitrophenyl derivatives, including this compound. The results indicated significant activity against a range of bacterial strains, highlighting its potential as a lead compound for new antibiotic formulations .
Q & A
Q. What are the key considerations for optimizing the synthesis of 4-(2-nitrophenyl)butyronitrile via decarboxylation reactions?
The synthesis can be optimized by controlling reaction parameters such as temperature, base concentration, and solvent selection. For example, intermediates like 4-(3-methyl-5-nitropyrid-2-yl)-4,4-bis(ethoxycarbonyl)butyronitrile undergo decarboxylation using NaOH in ethanol-water under reflux. Monitoring reaction progress via TLC or HPLC ensures complete conversion, while purification through recrystallization or column chromatography enhances purity . Kinetic studies under inert atmospheres (e.g., N₂) reduce side reactions, and stoichiometric optimization minimizes byproduct formation.
Q. How can researchers address challenges in detecting this compound in biological matrices using LC-MS?
Detection in complex biological samples requires solid-phase extraction (SPE) to remove interferents. Deuterated internal standards (e.g., AOZ-d4, AMOZ-d5) improve quantification accuracy by compensating for matrix effects and ionization variability . Method validation should include linearity (R² > 0.99), limits of detection (LOD < 1 ppb), and recovery rates (80–120%) across pH and ionic strength ranges. Post-column infusion experiments identify ion suppression zones, guiding mobile phase optimization.
Q. What spectroscopic techniques are most effective for confirming the structural integrity of this compound derivatives?
Multi-nuclear NMR (¹H, ¹³C) resolves nitrophenyl and nitrile group assignments, with chemical shifts for C≡N typically near 120 ppm in ¹³C spectra. IR spectroscopy confirms C≡N stretching (~2240 cm⁻¹) and nitro group vibrations (1520–1350 cm⁻¹). High-resolution mass spectrometry (HRMS) provides exact mass confirmation (e.g., [M+H]⁺ calculated vs. observed), while X-ray crystallography resolves stereochemical ambiguities in crystalline derivatives .
Q. What experimental strategies mitigate data discrepancies when analyzing reaction intermediates of this compound?
Discrepancies often arise from impurities or competing pathways. Orthogonal methods (e.g., HPLC paired with GC-MS) cross-validate results. Statistical analysis of triplicate replicates identifies outliers, and kinetic profiling under controlled conditions (e.g., 25°C ± 0.5°C) reduces variability. Certified reference materials (CRMs) and spike-recovery experiments (90–110% recovery) validate analytical accuracy .
Q. How does the electron-withdrawing nitro group influence the chemical reactivity of this compound in nucleophilic substitutions?
The ortho-nitro group activates the nitrile toward nucleophilic attack via electron withdrawal, facilitating reactions with amines or thiols to form amides or thioethers. Steric hindrance from the nitro group may direct regioselectivity, necessitating polar aprotic solvents (e.g., DMF) and catalysts (e.g., K₂CO₃) to enhance yields. Computational modeling (DFT) predicts transition states, guiding solvent and catalyst selection .
Q. What are the best practices for handling and storing this compound to prevent degradation during long-term studies?
Store in amber-glass containers under inert gas (N₂/Ar) at 2–8°C to mitigate hydrolysis and photodegradation. Regular purity checks via HPLC (≥98% purity) or ¹H NMR ensure stability. Grounding containers during transfers prevents static discharge, and explosion-proof equipment is mandatory in handling areas .
Q. How can computational chemistry aid in predicting the stability of this compound under varying experimental conditions?
Density Functional Theory (DFT) calculates bond dissociation energies (BDEs) and reaction pathways, identifying vulnerable sites (e.g., nitrile group). Molecular dynamics simulations model solvation effects, predicting stability in solvents like acetonitrile or THF. Thermogravimetric analysis (TGA) validates computational predictions of thermal decomposition thresholds .
Q. What methodological approaches resolve contradictions in reaction yields reported for this compound synthesis?
Yield discrepancies often stem from unoptimized workup or characterization methods. Replicating procedures with strict control of moisture and oxygen levels isolates variables. Comparative studies using alternative purification techniques (e.g., distillation vs. chromatography) quantify efficiency. Meta-analyses of published protocols identify consensus conditions (e.g., 70°C, 12h reaction time) .
Comparison with Similar Compounds
Comparison with Structural Analogs
Positional Isomers: Nitrophenyl Substitution
- 4-(4-Nitrophenyl)butyronitrile (C₁₀H₁₀N₂O₂): Molecular weight: 190.199 g/mol. However, the electronic effects of the nitro group (meta-directing) differ, altering reactivity in electrophilic substitution reactions .
- 2-(3-Nitrophenyl)-4-oxo-4-phenylbutanenitrile (C₁₆H₁₂N₂O₃) :
Key Difference : The ortho-nitro group in 4-(2-Nitrophenyl)butyronitrile induces steric strain, favoring intramolecular cyclization over para/meta isomers .
Functional Group Variations
4-(4-Nitrophenyl)butyric Acid (C₁₀H₁₁NO₄)
- Molecular weight: 209.20 g/mol.
- Replaces the nitrile with a carboxylic acid (-COOH), increasing polarity and enabling salt formation. Used in biochemical assays due to its UV-active nitro group .
4-(Dimethylamino)butyronitrile Hydrochloride (C₆H₁₂N₂·HCl)
- Molecular weight: 148.63 g/mol.
- The dimethylamino group (-N(CH₃)₂) enhances nucleophilicity, making it suitable for alkylation reactions. The hydrochloride salt improves stability and solubility in aqueous media .
Key Difference: The nitrile group in this compound facilitates nucleophilic additions (e.g., hydrolysis to amides), unlike carboxylic acid or amino derivatives .
Hydroxyl-Substituted Derivatives
2-(4-Hydroxy-3-nitrophenyl)butyronitrile (C₁₀H₁₀N₂O₃)
- Molecular weight: 206.20 g/mol.
- Boiling point: 329.1°C; Density: 1.291 g/cm³.
- The hydroxyl group adjacent to the nitro substituent enables hydrogen bonding, increasing melting/boiling points compared to non-hydroxylated analogs.
Key Difference : Hydroxyl groups introduce additional reactivity (e.g., oxidation or conjugation), absent in this compound .
Substituent Effects on Reactivity
- 4-(2-Iodophenoxy)butyronitrile and 4-(2-Formylphenoxy)butyronitrile: Iodo and formyl substituents enable diverse reactivity. The iodo group acts as a leaving group in nucleophilic substitutions, while the formyl group participates in condensations (e.g., forming Schiff bases) .
- N-(4-Dimethylamino-3,5-dinitrophenyl)acetonitrile: Contains dual nitro groups and a dimethylamino substituent, enhancing electron-withdrawing effects. Such compounds are explored as enzyme inhibitors or fluorescent probes .
Key Difference : The ortho-nitro group in this compound directs cyclization reactions, whereas electron-donating groups (e.g., -OCH₃) or bulky substituents alter reaction pathways .
Data Tables
Table 1: Physical Properties of Selected Nitriles
*Estimated based on structural similarity.
Preparation Methods
Base-Catalyzed Condensation of 2-Nitrotoluene and Acrylonitrile
The most widely documented method for synthesizing 4-(2-nitrophenyl)butyronitrile involves the condensation of 2-nitrotoluene with acrylonitrile in the presence of a strong base and an aprotic polar solvent. This reaction proceeds via a Michael addition mechanism, where the base deprotonates the nitrophenylalkane to generate a carbanion, which subsequently attacks the electron-deficient β-carbon of acrylonitrile .
Reaction Conditions and Catalysts
Key parameters include:
-
Catalyst : Alkali metal hydroxides (e.g., NaOH, KOH) or quaternary ammonium hydroxides (e.g., tetramethylammonium hydroxide) at 1–5 wt% relative to 2-nitrotoluene .
-
Solvent : Polar aprotic solvents such as dimethylformamide (DMF), hexamethylphosphoramide, or N-acetylmorpholine. DMF is preferred due to its high dielectric constant and ability to stabilize ionic intermediates .
-
Temperature : 0–50°C, with optimal yields observed at 25–30°C .
-
Atmosphere : Inert gas (e.g., nitrogen or argon) to prevent oxidation of intermediates .
Table 1: Representative Reaction Parameters from Patent Examples
Workup and Purification
Post-reaction, the mixture is neutralized with dilute hydrochloric acid, followed by solvent removal under reduced pressure. The crude product is purified via distillation (for liquid nitriles) or recrystallization from petroleum ether . Spectral characterization (IR, NMR) confirms the presence of nitrile (ν~2240 cm⁻¹) and nitro (ν~1520, 1350 cm⁻¹) functional groups .
Alternative Synthetic Routes and Modifications
Substituent Variations
The patent literature demonstrates flexibility in modifying the nitrophenyl or acrylonitrile components:
-
Alkyl-Substituted Acrylonitriles : Methacrylonitrile yields 2-methyl-4-(2-nitrophenyl)butyronitrile, though steric effects reduce yields by 10–15% compared to unsubstituted acrylonitrile .
-
Di-Nitro Substrates : 2,4-Dinitrotoluene reacts similarly but requires extended reaction times (4–6 hr) due to electronic deactivation .
Solvent Optimization
Non-polar co-solvents (e.g., toluene) are occasionally added to improve miscibility of aromatic substrates, though this may necessitate higher catalyst loadings .
Industrial-Scale Considerations
Economic Viability
The process is cost-effective due to:
-
Low catalyst loading (≤5 wt%).
-
Commodity-priced starting materials (2-nitrotoluene: ~$15/kg; acrylonitrile: ~$2/kg).
Applications in Downstream Synthesis
This compound serves as a precursor for:
Properties
Molecular Formula |
C10H10N2O2 |
---|---|
Molecular Weight |
190.20 g/mol |
IUPAC Name |
4-(2-nitrophenyl)butanenitrile |
InChI |
InChI=1S/C10H10N2O2/c11-8-4-3-6-9-5-1-2-7-10(9)12(13)14/h1-2,5,7H,3-4,6H2 |
InChI Key |
WTLIFYPMYIVCCH-UHFFFAOYSA-N |
Canonical SMILES |
C1=CC=C(C(=C1)CCCC#N)[N+](=O)[O-] |
Origin of Product |
United States |
Synthesis routes and methods I
Procedure details
Synthesis routes and methods II
Procedure details
Synthesis routes and methods III
Procedure details
Disclaimer and Information on In-Vitro Research Products
Please be aware that all articles and product information presented on BenchChem are intended solely for informational purposes. The products available for purchase on BenchChem are specifically designed for in-vitro studies, which are conducted outside of living organisms. In-vitro studies, derived from the Latin term "in glass," involve experiments performed in controlled laboratory settings using cells or tissues. It is important to note that these products are not categorized as medicines or drugs, and they have not received approval from the FDA for the prevention, treatment, or cure of any medical condition, ailment, or disease. We must emphasize that any form of bodily introduction of these products into humans or animals is strictly prohibited by law. It is essential to adhere to these guidelines to ensure compliance with legal and ethical standards in research and experimentation.