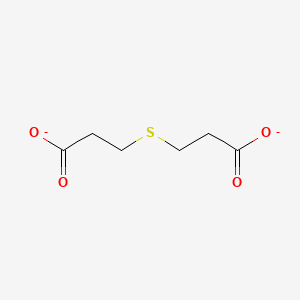
3,3'-Thiodipropanoate
- Click on QUICK INQUIRY to receive a quote from our team of experts.
- With the quality product at a COMPETITIVE price, you can focus more on your research.
Overview
Description
3,3'-Thiodipropanoate is a sulfur-containing organic compound characterized by two propanoate groups linked via a thioether (-S-) bond. Its derivatives, particularly esters such as dimethyl, diethyl, dilauryl, and dioctadecyl esters, are widely used as antioxidants in polymers, stabilizers in plastics, and intermediates in pharmaceuticals. These compounds function by scavenging free radicals, thereby inhibiting oxidative degradation in materials .
The general structure of this compound esters is shown below: R-O-CO-CH2-CH2-S-CH2-CH2-CO-O-R where R represents alkyl groups of varying chain lengths (e.g., methyl, ethyl, lauryl, stearyl). The choice of the alkyl chain significantly influences physical properties, solubility, and industrial applications.
Scientific Research Applications
Scientific Research Applications
-
Biochemical Research :
- Metabolic Pathways : Research indicates that certain bacteria, such as Variovorax paradoxus, can utilize 3,3'-thiodipropanoate as a carbon source, leading to the production of 3-mercaptopropionate, a precursor for polythioesters. This pathway has implications for biopolymer synthesis and metabolic engineering .
- Antioxidant Properties : Studies have demonstrated that compounds like dimethyl this compound exhibit antioxidant activities by scavenging free radicals, which can be pivotal in preventing oxidative stress-related diseases .
-
Material Science :
- Polymer Production : Thiodipropanoates serve as stabilizers and antioxidants in polymer formulations. For instance, dilauryl thiodipropionate is utilized in polyethylene and polypropylene films to enhance thermal stability and prolong shelf life .
- Coatings and Plastics : The incorporation of thiodipropanoates into coatings improves their durability against environmental factors, making them valuable in industrial applications.
-
Pharmaceutical Applications :
- Drug Delivery Systems : The unique chemical properties of thiodipropanoates allow them to be explored as carriers in drug delivery systems. Their ability to form stable complexes with various drugs enhances bioavailability and targeted delivery.
- Anticancer Research : Thiodipropanoates have been investigated for their potential in cancer therapies due to their ability to modify cellular interactions and stabilize protein complexes involved in tumor growth .
Case Studies
Study | Findings |
---|---|
Sahu et al. (2023) | Investigated the antioxidant properties of dimethyl this compound, confirming its efficacy in scavenging free radicals and preventing oxidative stress. |
Paprocka et al. (2023) | Demonstrated anti-inflammatory effects of related thioester compounds, suggesting therapeutic potential for this compound derivatives in inflammatory diseases. |
Ekiert and Szopa (2023) | Explored the synthesis of oxygenated derivatives of similar compounds, revealing significant antimicrobial and anticancer activities parallel to those observed with thiodipropanoates. |
Industrial Applications
- Food Industry : Thiodipropanoates are recognized for their safety and efficacy as antioxidants in food packaging materials, contributing to food preservation by inhibiting oxidation processes .
- Cosmetics : In cosmetic formulations, dilauryl thiodipropionate has been assessed for skin compatibility and safety, showing no adverse effects under standard testing conditions .
Chemical Reactions Analysis
Hydrolysis and Stability
The ester groups in 3,3'-thiodipropanoate derivatives hydrolyze in aqueous environments to form 3,3'-thiodipropionic acid (TDPA). This reaction is critical in environmental and biological systems, where esterases or acidic/basic conditions catalyze the conversion .
DMTDP+2H2O→TDPA+2CH3OH
Biological Degradation in Variovorax paradoxus
Variovorax paradoxus strain TBEA6 metabolizes TDPA via a specialized pathway involving enzymatic cleavage and CoA-dependent intermediates :
Key Steps in TDPA Catabolism:
-
Initial Cleavage :
TDPA is cleaved into 3-mercaptopropionate (3MP) via a putative enoyl-CoA hydratase (Ech-20), which acts on TDP-CoA . -
Oxidation :
3MP is oxidized to 3-sulfinopropionate (3SP) by a cysteine dioxygenase-like enzyme (Mdo) . -
CoA Activation :
3SP is converted to 3SP-CoA via succinyl-CoA transferase (SucCD) . -
Desulfination :
3SP-CoA is cleaved into propionyl-CoA and sulfite by acyl-CoA dehydrogenase (AcdA) .
Enzyme | Function | Substrate | Product |
---|---|---|---|
Ech-20 | TDP-CoA cleavage | TDP-CoA | 3MP + CoA |
Mdo | 3MP oxidation | 3MP | 3SP |
SucCD | CoA activation | 3SP | 3SP-CoA |
AcdA | Desulfination | 3SP-CoA | Propionyl-CoA + SO₃²⁻ |
Side Reactions and By-Product Formation
During industrial synthesis, competing reactions generate undesired by-products:
-
Methyl-β-mercaptopropionate : Forms via incomplete Michael addition .
-
Polymerization : Occurs at elevated temperatures or with residual acrylate monomers .
-
Diekmann Cyclization : Produces cyclic esters under basic conditions .
Metabolic Fate in Mammalian Systems
In rats, orally administered TDPA is partially excreted unchanged in urine (14% of dose), while the remainder undergoes uncharacterized metabolic transformations, likely involving gut microbiota or hepatic enzymes .
Q & A
Basic Research Questions
Q. Q1. What experimental methodologies are most effective for synthesizing and characterizing 3,3'-Thiodipropanoate in a laboratory setting?
Methodological Answer:
- Synthesis Optimization : Use controlled esterification or thiol-disulfide exchange reactions under inert atmospheres (e.g., nitrogen) to minimize oxidation byproducts. Monitor reaction progress via thin-layer chromatography (TLC) or HPLC .
- Characterization Techniques : Employ nuclear magnetic resonance (NMR) spectroscopy (¹H and ¹³C) for structural elucidation, Fourier-transform infrared spectroscopy (FTIR) to confirm functional groups (e.g., thioether bonds), and mass spectrometry (MS) for molecular weight validation. Thermal stability can be assessed via differential scanning calorimetry (DSC) .
- Data Interpretation : Cross-reference spectral data with computational simulations (e.g., density functional theory) to resolve ambiguities in peak assignments .
Q. Q2. How can researchers address inconsistencies in reported solubility and stability data for this compound across different solvents?
Methodological Answer:
- Controlled Replication : Standardize solvent purity, temperature (±0.5°C), and agitation methods (e.g., orbital shaker vs. magnetic stirring) to isolate variables influencing solubility .
- Advanced Analytical Tools : Use dynamic light scattering (DLS) to monitor aggregation in polar solvents (e.g., water, ethanol) and gas chromatography (GC) to detect degradation byproducts under acidic/alkaline conditions .
- Statistical Validation : Apply ANOVA or regression models to quantify the impact of solvent polarity and pH on stability, ensuring sample sizes (n ≥ 3) meet statistical power requirements .
Advanced Research Questions
Q. Q3. What mechanistic pathways govern the reactivity of this compound in redox-active environments, and how can these be experimentally validated?
Methodological Answer:
- Hypothesis Testing : Propose competing pathways (e.g., radical-mediated vs. nucleophilic substitution) and design isotope-labeling experiments (e.g., deuterated solvents) to track hydrogen abstraction or sulfur-centered radical formation .
- In Situ Monitoring : Utilize electron paramagnetic resonance (EPR) spectroscopy to detect transient radicals, coupled with cyclic voltammetry to map redox potentials .
- Contradiction Resolution : Compare kinetic data (e.g., rate constants) across pH gradients and correlate with computational models (e.g., Marcus theory) to reconcile conflicting mechanistic proposals .
Q. Q4. How can computational modeling (e.g., molecular dynamics, QSAR) predict the biological interactions of this compound with enzymatic targets?
Methodological Answer:
- Model Selection : Use molecular docking (AutoDock Vina) to screen binding affinities with sulfhydryl-active enzymes (e.g., glutathione reductase). Validate predictions via in vitro enzyme inhibition assays .
- Data Integration : Cross-reference Quantitative Structure-Activity Relationship (QSAR) predictions with experimental IC₅₀ values to refine model accuracy. Address discrepancies by adjusting force field parameters (e.g., AMBER vs. CHARMM) .
- Ethical Considerations : Ensure alignment with institutional guidelines for in silico-to-in vivo extrapolation, particularly for toxicity predictions .
Q. Q5. What strategies mitigate confounding variables in cross-disciplinary studies involving this compound (e.g., materials science and toxicology)?
Methodological Answer:
- Interdisciplinary Frameworks : Establish standardized protocols for sample preparation (e.g., solvent-free synthesis for biocompatibility assays) to ensure consistency across disciplines .
- Blinded Experiments : Implement double-blinded toxicity screenings (e.g., cell viability assays) to reduce bias in data interpretation .
- Meta-Analysis : Aggregate data from heterogeneous studies using PRISMA guidelines to identify systemic trends or outliers in reported outcomes .
Q. Key Recommendations for Researchers
- Literature Review : Prioritize peer-reviewed journals over commercial databases (e.g., PubMed, SciFinder) to avoid unreliable sources .
- Ethical Compliance : Adhere to institutional safety protocols for handling thioether compounds, including PPE and fume hood use .
- Data Transparency : Archive raw datasets in repositories like Zenodo to facilitate reproducibility .
Comparison with Similar Compounds
Structural and Physical Properties
The table below compares key physical properties of 3,3'-thiodipropanoate derivatives:
Key Observations :
- Chain Length and Boiling Point : Longer alkyl chains (e.g., dioctadecyl) increase molecular weight and boiling point due to stronger van der Waals interactions .
- Solubility : Shorter-chain esters (e.g., dimethyl) are more polar and soluble in organic solvents, whereas longer-chain derivatives (e.g., dilauryl) are lipophilic and compatible with polymers .
- Thermal Stability : Higher flash points in long-chain esters (e.g., dioctadecyl at 323.7°C) make them suitable for high-temperature processing .
Preparation Methods
Esterification of Thiodipropionic Acid
The most common method for synthesizing TDPA esters involves the direct esterification of 3,3'-thiodipropionic acid (TDPA) with alcohols. This route is widely adopted due to its scalability and compatibility with diverse alcohols, such as methanol, tridecanol, and stearyl alcohol.
Acid-Catalyzed Esterification
Sulfuric acid (H₂SO₄) and p-toluenesulfonic acid (PTSA) are the primary catalysts for this reaction. For example, the synthesis of dimethyl 3,3'-thiodipropionate (CAS 4131-74-2) involves refluxing TDPA with methanol in the presence of concentrated H₂SO₄ at 65–75°C for 4–6 hours . The water generated during the reaction is removed via azeotropic distillation to shift the equilibrium toward ester formation.
Key Reaction Conditions:
Parameter | Value |
---|---|
Molar Ratio (Acid:Alcohol) | 1:2.1–1:2.5 |
Catalyst Loading | 1–3 wt% (H₂SO₄) |
Temperature | 65–75°C |
Yield | 85–92% |
Enzymatic Esterification
Lipase B from Candida antarctica (CALB) offers a greener alternative for synthesizing TDPA esters. CALB catalyzes the transesterification of TDPA with fatty alcohols in non-aqueous media. For instance, distearyl 3,3'-thiodipropionate (CAS 693-36-7) is produced by reacting TDPA with stearyl alcohol at 60°C using immobilized CALB, achieving yields of 78–84% . Enzymatic methods reduce energy consumption and avoid corrosive catalysts but require longer reaction times (24–48 hours).
Hydrolysis of 3,3'-Thiodipropionitrile
3,3'-Thiodipropionitrile serves as a precursor for TDPA through controlled acid hydrolysis. This method is favored for its high atom economy and minimal byproduct formation.
Mineral Acid Hydrolysis
In a patented process, thiodipropionitrile is gradually added to a heated (70–120°C) aqueous solution of sulfuric acid (3–10 M) to maintain the nitrile concentration below 5 wt%, preventing exothermic runaway reactions . The hydrolysis proceeds via intermediate amide formation, culminating in TDPA after 4–6 hours.
Optimized Parameters:
Parameter | Value |
---|---|
H₂SO₄ Concentration | 6–8 M |
Reaction Time | 4–6 hours |
Yield | 88–95% |
Alkaline Hydrolysis
Alkaline conditions using NaOH or KOH (2–5 M) at 80–100°C yield TDPA salts, which are acidified to isolate the free acid. However, this method generates stoichiometric amounts of inorganic waste, limiting its industrial appeal .
Thiol-Ene Reaction for Thiodipropionate Esters
The thiol-ene reaction between mercaptans and α,β-unsaturated esters provides a versatile route to asymmetric TDPA derivatives. For example, dilauryl thiodipropionate (CAS 123-28-4) is synthesized by reacting lauryl mercaptan with methyl acrylate in the presence of radical initiators like azobisisobutyronitrile (AIBN) .
Reaction Mechanism:
-
Initiation : AIBN decomposes to generate radicals.
-
Propagation : Radicals abstract hydrogen from the mercaptan, forming thiyl radicals.
-
Addition : Thiyl radicals add to the acrylate double bond, forming C–S bonds.
Typical Conditions:
Parameter | Value |
---|---|
Temperature | 70–90°C |
Initiator Loading | 0.5–1 mol% AIBN |
Yield | 75–82% |
Biocatalytic Synthesis
Microbial pathways offer emerging routes for TDPA production. Variovorax paradoxus TBEA6 metabolizes 3,3'-thiodipropionitrile via a two-step enzymatic cascade:
-
Nitrilase : Converts nitrile to 3-mercaptopropionic acid (3MP).
Advantages:
-
Operates under mild conditions (30–37°C, pH 7–8).
-
Eliminates toxic solvents.
Challenges:
-
Low volumetric productivity (0.1–0.5 g/L·h).
-
Requires genetic engineering for scale-up.
Industrial-Scale Optimization
Continuous Flow Reactors
Recent patents describe continuous esterification systems using packed-bed reactors with solid acid catalysts (e.g., sulfonated zirconia). These systems achieve 95% conversion in 30 minutes at 120°C, reducing energy costs by 40% compared to batch processes .
Solvent-Free Synthesis
Molten TDPA and alcohols react without solvents at 130–150°C, enhancing reaction rates and eliminating VOC emissions. For example, ditridecyl thiodipropionate (CAS 10595-72-9) is produced with 94% yield using this approach.
Comparative Analysis of Methods
Method | Yield (%) | Energy Use | Scalability | Environmental Impact |
---|---|---|---|---|
Acid-Catalyzed Esterification | 85–92 | High | Excellent | Moderate (acid waste) |
Enzymatic Hydrolysis | 78–84 | Low | Moderate | Low |
Thiol-Ene Reaction | 75–82 | Moderate | Good | High (VOC emissions) |
Biocatalytic | 60–70 | Very Low | Limited | Very Low |
Properties
Molecular Formula |
C6H8O4S-2 |
---|---|
Molecular Weight |
176.19 g/mol |
IUPAC Name |
3-(2-carboxylatoethylsulfanyl)propanoate |
InChI |
InChI=1S/C6H10O4S/c7-5(8)1-3-11-4-2-6(9)10/h1-4H2,(H,7,8)(H,9,10)/p-2 |
InChI Key |
ODJQKYXPKWQWNK-UHFFFAOYSA-L |
Canonical SMILES |
C(CSCCC(=O)[O-])C(=O)[O-] |
Origin of Product |
United States |
Disclaimer and Information on In-Vitro Research Products
Please be aware that all articles and product information presented on BenchChem are intended solely for informational purposes. The products available for purchase on BenchChem are specifically designed for in-vitro studies, which are conducted outside of living organisms. In-vitro studies, derived from the Latin term "in glass," involve experiments performed in controlled laboratory settings using cells or tissues. It is important to note that these products are not categorized as medicines or drugs, and they have not received approval from the FDA for the prevention, treatment, or cure of any medical condition, ailment, or disease. We must emphasize that any form of bodily introduction of these products into humans or animals is strictly prohibited by law. It is essential to adhere to these guidelines to ensure compliance with legal and ethical standards in research and experimentation.