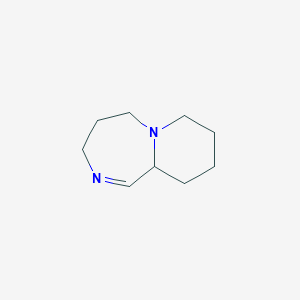
1,5-Diazabicyclo(5,4,0)undec-5-ene
Overview
Description
1,5-Diazabicyclo[5.4.0]undec-5-ene (DBU) is a bicyclic amidine characterized by an 11-membered ring system with nitrogen atoms at positions 1 and 5 (bridged bicyclo[5.4.0] framework). It is a potent, non-nucleophilic organic base (pKa ~12–13 in water) widely employed in organic synthesis, polymerization catalysis, and dehydrohalogenation reactions . Key applications include:
- Ring-Opening Polymerization (ROP): DBU catalyzes the ROP of lactones and cyclic carbonates, enabling rapid synthesis of polyesters and polycarbonates with controlled molecular weights .
- Metal-Free Synthesis: Its use avoids toxic metal catalysts (e.g., stannous octoate), making it ideal for biomedical polymer synthesis .
- Oxidation Reactions: DBU enhances efficiency in Cu-catalyzed aerobic oxidations, outperforming other bases like DBN and DMAP .
Preparation Methods
Synthetic Routes Derived from Bicyclic Amidine Precursors
The synthesis of bicyclic amidines typically involves multi-step reactions starting from lactams or cyclic amines. For 1,5-diazabicyclo(5,4,0)undec-5-ene, the most feasible pathway appears to mirror the synthesis of DBU and DBN, which employ caprolactam or pyrrolidone as starting materials .
Michael Addition of Cyclic Amines to Acrylonitrile
A critical first step involves the Michael addition of a cyclic amine to acrylonitrile. For DBU synthesis, caprolactam reacts with acrylonitrile to form N-(2-cyanoethyl)-caprolactam . By analogy, 2-piperidone or a similar seven-membered lactam could serve as the starting material for this compound. The reaction is typically catalyzed by a base such as sodium hydroxide (NaOH) in a solvent like tert-butyl alcohol .
Reaction Conditions:
Hydrogenation of Cyano Intermediate
The cyano group in the Michael adduct is hydrogenated to an amine. For DBU, this step converts N-(2-cyanoethyl)-caprolactam to N-aminopropyl-caprolactam using hydrogen gas and a Raney nickel catalyst . Similar conditions would apply to the this compound precursor.
Key Parameters:
-
Pressure: 3–5 bar H₂.
-
Catalyst: Raney nickel or palladium on carbon.
-
Solvent: Retained from the previous step (no solvent removal) .
Cyclization via Dehydration
The final step involves intramolecular cyclization to form the bicyclic amidine structure. Heating the amine intermediate with a dehydrating agent (e.g., phosphorus oxychloride or thionyl chloride) facilitates imine formation . For DBU, this step achieves 80% yield under optimized conditions .
Optimization Insights:
Comparative Analysis of Synthetic Efficiency
The synthesis of this compound faces challenges similar to those encountered in DBU production, including low cyclization yields and solvent compatibility. The table below contrasts reported data for DBU with inferred parameters for this compound:
Solvent and Catalyst Optimization
The choice of solvent and catalyst significantly impacts reaction efficiency. Tertiary alcohols like tert-butyl alcohol enhance Michael addition kinetics by stabilizing the transition state . Catalysts such as NaOH or KOH are preferred for their low cost and high activity in promoting cyanoethylation .
Catalyst Load Study:
-
Excess catalyst (>2 mol%) may induce side reactions, reducing yield.
Challenges in Purification and Isolation
Post-cyclization purification often requires fractional distillation under reduced pressure. For DBU, boiling points range from 83°C (at 1 mmHg) to 116°C (ambient pressure) . The this compound likely exhibits similar volatility, necessitating precise temperature control to prevent decomposition.
Purification Data:
-
Projected Purity for 1,5-Isomer: 90–95% without advanced chromatography.
Alternative Pathways and Novel Approaches
Recent advances in organocatalysis suggest potential for using this compound as a catalyst in its own synthesis. For example, 1,5,7-triazabicyclo[4.4.0]dec-5-ene (TBD) has been employed in amidation–cyclization cascades , hinting at opportunities for intramolecular catalysis.
Mechanistic Considerations:
-
Base-mediated dehydrohalogenation.
-
Lewis acid-assisted cyclization.
Industrial-Scale Production Considerations
Scaling up the synthesis requires addressing solvent recovery and catalyst recycling. The patent CN101279973A highlights solvent reuse in DBU production, reducing material costs by 15–20% . Similar strategies could be applied to the 1,5-isomer.
Cost Drivers:
-
Raw Materials: ~60% of total cost.
-
Energy: ~25% (distillation dominates).
Chemical Reactions Analysis
Elimination Reactions
1,5-Diazabicyclo(5,4,0)undec-5-ene acts as a base catalyst in dehydrohalogenation reactions, facilitating the removal of halides (e.g., HX) to form alkenes. This process is widely used in the synthesis of olefins.
Example :
-
Substrate : Alkyl halides (e.g., bromoalkanes).
-
Conditions : Basic medium.
Aldol Condensation
The compound catalyzes the Aldol condensation, a reaction between carbonyl compounds and enolates to form β-hydroxy ketones. This reaction is fundamental in organic synthesis for constructing complex molecules.
Example :
-
Substrate : Carbonyl compounds (e.g., aldehydes, ketones).
-
Conditions : Basic medium.
Epimerization
This compound facilitates the epimerization of penicillin derivatives, a critical step in modifying the stereochemistry of β-lactam antibiotics.
Example :
-
Substrate : Penicillin derivatives.
-
Conditions : Basic medium.
Comparative Analysis of Reaction Types
Reaction Type | Key Features | Applications |
---|---|---|
Elimination | Base-catalyzed dehydrohalogenation | Olefin synthesis |
Aldol Condensation | Formation of β-hydroxy ketones | Construction of complex molecules |
Epimerization | Stereochemical rearrangement | Modification of penicillin derivatives |
Ionic Liquid Use | Solvent replacement in cellulose processing | Lyocell production |
Scientific Research Applications
Scientific Research Applications
-
Organic Synthesis
- Catalyst in Reactions : Utilized as a catalyst in various organic reactions such as the Baylis-Hillman reaction and aza-Michael addition.
- Base for Dehydrohalogenation : Acts as a strong base to facilitate the removal of halogen atoms from organic compounds.
- Esterification : Catalyzes the esterification of carboxylic acids with alcohols.
-
Polymer Chemistry
- Curing Agent : Employed in the curing processes of epoxy resins and polyurethane, enhancing the mechanical properties of these materials.
-
Pharmaceuticals
- Synthesis of Intermediates : Used in the synthesis of pharmaceutical intermediates and active pharmaceutical ingredients, contributing to drug development.
-
Material Science
- Nanomaterials Preparation : Involved in the preparation of advanced materials, including fullerenes and other nanostructures.
Case Studies
-
Baylis-Hillman Reaction
- In a study published in the Journal of Medicinal and Chemical Sciences, 1,5-Diazabicyclo(5,4,0)undec-5-ene was shown to effectively catalyze the Baylis-Hillman reaction between aldehydes and α,β-unsaturated carbonyl compounds. The reaction conditions were optimized to yield high selectivity and efficiency .
- Antimicrobial Activity
-
Polymer Applications
- Research highlighted the use of this compound as a curing agent for epoxy resins. The resulting materials exhibited enhanced thermal stability and mechanical strength compared to traditional curing agents .
Mechanism of Action
The mechanism of action of 1,5-Diazabicyclo(5,4,0)undec-5-ene involves its strong basicity and ability to act as a proton acceptor. It facilitates various reactions by deprotonating substrates, thereby increasing their nucleophilicity or reactivity. The compound’s bicyclic structure provides steric hindrance, preventing it from participating directly in nucleophilic attacks .
Comparison with Similar Compounds
Comparison with Structurally Similar Compounds
Structural and Basicity Differences
DBU is frequently compared to two other bicyclic amidines: 1,5-Diazabicyclo[4.3.0]non-5-ene (DBN) and 1,5,7-Triazabicyclo[4.4.0]dec-5-ene (TBD).
Note: DBU’s larger ring size confers greater basicity compared to DBN. TBD, with an additional nitrogen, exhibits the highest basicity and catalytic activity in ROP .
Ring-Opening Polymerization (ROP)
- DBU: Efficient for lactones and cyclic carbonates; produces polymers with low dispersity (Đ <1.2) in minutes .
- TBD: Faster polymerization than DBU due to higher nucleophilicity; achieves similar molecular weight control .
- DBN: Less effective in ROP compared to DBU and TBD, primarily used in dehydrohalogenation .
Dehydrohalogenation
- DBN: Superior to pyridine and morpholine derivatives in synthesizing α,β-unsaturated acetylenes (e.g., Vitamin A acetate).
- DBU: Comparable reactivity but preferred for thermally sensitive substrates due to milder conditions .
Oxidation Catalysis
In CuBr₂-catalyzed oxidations of 3,4-dihydro-β-carbolines, DBU outperformed DBN and DMAP, achieving >90% yield with 2.0 equivalents .
Thermochemical Properties
DBU and DBN exhibit higher vaporization enthalpies (ΔvapH) compared to simpler heterocycles (e.g., pyridine derivatives) due to strong self-association via hydrogen bonding . This property influences their use in high-temperature reactions and distillation processes.
Q & A
Basic Research Questions
Q. How does DBU enhance catalytic efficiency in dehydrohalogenation reactions compared to traditional amines?
DBU’s bicyclic structure and strong basicity (pKa ~12.5) enable rapid proton abstraction under mild conditions, minimizing side reactions like nucleophilic substitution. Its non-nucleophilic nature prevents unwanted adduct formation, critical for synthesizing sensitive intermediates such as α,β-unsaturated acetylenes. For instance, DBU outperformed pyridine and γ,δ-dimethylaniline in Vitamin A acetate synthesis by avoiding decomposition pathways .
Q. What experimental parameters are critical when designing DBU-catalyzed Michael addition reactions?
Key factors include:
- Solvent selection : Polar aprotic solvents (e.g., THF, DMF) enhance DBU’s solubility and activity.
- Temperature control : Reactions often proceed at 25–60°C to balance kinetics and stability.
- Stoichiometry : Substrate-to-DBU ratios (typically 1:0.1–0.3 mol%) must be optimized to avoid overbasification, which can degrade sensitive electrophiles .
Q. How does DBU’s molecular structure influence its reactivity in ring-opening polymerizations?
DBU’s bicyclic framework stabilizes transition states via intramolecular hydrogen bonding, facilitating nucleophilic attack on cyclic esters (e.g., lactides). Its steric hindrance reduces chain-transfer reactions, enabling controlled polymer molecular weights. Comparative studies show DBU achieves higher polydispersity indices (<1.2) than DBN in PLA synthesis .
Advanced Research Questions
Q. How can kinetic studies resolve contradictions in DBU-mediated reaction mechanisms?
Conflicting reports on DBU’s role (base vs. nucleophile) in SNAr reactions require:
- In-situ monitoring : Use in operando NMR or IR spectroscopy to track intermediate formation.
- Isotopic labeling : Deuterated substrates can distinguish proton-transfer steps from covalent adducts.
- Computational modeling : DFT studies (e.g., COMSOL Multiphysics) elucidate transition states and confirm DBU’s non-nucleophilic behavior in specific systems .
Q. What methodologies validate DBU’s role in stabilizing reactive intermediates during multi-step syntheses?
- Trapping experiments : Add electrophilic quenchers (e.g., methyl triflate) to isolate intermediates.
- Crystallographic analysis : Single-crystal X-ray diffraction of DBU-coordinated species (e.g., enolates) confirms structural stabilization.
- Mass spectrometry : HRMS identifies transient intermediates in flow-chemistry setups .
Q. How do solvent and moisture levels affect reproducibility in DBU-catalyzed reactions?
DBU’s hygroscopicity necessitates rigorous drying protocols (e.g., molecular sieves, inert atmosphere). Water hydrolyzes DBU to form urea derivatives, reducing catalytic activity. Solvent choice (e.g., anhydrous DCM vs. wet THF) impacts reaction rates by 30–50% in esterifications, as shown by DoE (Design of Experiments) factorial analyses .
Q. Data Analysis and Optimization
Q. What statistical approaches address variability in DBU-mediated reaction yields?
- Factorial Design : Screen variables (temperature, catalyst loading) to identify dominant factors.
- Response Surface Methodology (RSM) : Optimize conditions for maximum yield.
- ANOVA : Quantify significance of interactions (e.g., solvent polarity × DBU concentration) .
Q. How can computational tools predict DBU’s compatibility with novel substrates?
- Molecular docking : Simulate DBU-substrate interactions using software like AutoDock Vina.
- pKa matching : Databases (e.g., Reaxys) compare substrate acidity with DBU’s basicity to forecast protonation feasibility.
- Machine learning : Train models on existing reaction datasets to recommend optimal conditions .
Q. Safety and Handling
Q. What PPE and waste management protocols are essential for DBU use?
Properties
CAS No. |
41015-70-7 |
---|---|
Molecular Formula |
C9H16N2 |
Molecular Weight |
152.24 g/mol |
IUPAC Name |
3,4,5,7,8,9,10,10a-octahydropyrido[1,2-a][1,4]diazepine |
InChI |
InChI=1S/C9H16N2/c1-2-6-11-7-3-5-10-8-9(11)4-1/h8-9H,1-7H2 |
InChI Key |
KWPQTFXULUUCGD-UHFFFAOYSA-N |
Canonical SMILES |
C1CCN2CCCN=CC2C1 |
Origin of Product |
United States |
Synthesis routes and methods
Procedure details
Disclaimer and Information on In-Vitro Research Products
Please be aware that all articles and product information presented on BenchChem are intended solely for informational purposes. The products available for purchase on BenchChem are specifically designed for in-vitro studies, which are conducted outside of living organisms. In-vitro studies, derived from the Latin term "in glass," involve experiments performed in controlled laboratory settings using cells or tissues. It is important to note that these products are not categorized as medicines or drugs, and they have not received approval from the FDA for the prevention, treatment, or cure of any medical condition, ailment, or disease. We must emphasize that any form of bodily introduction of these products into humans or animals is strictly prohibited by law. It is essential to adhere to these guidelines to ensure compliance with legal and ethical standards in research and experimentation.