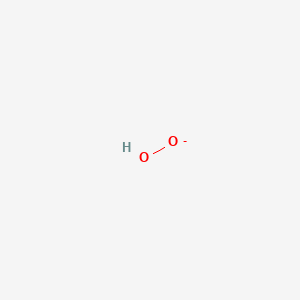
Hydrogenperoxide(1-)
Overview
Description
Hydrogen peroxide (H₂O₂), a simple peroxide with the chemical formula H₂O₂, is a pale blue liquid in pure form, though it is typically handled as an aqueous solution due to its instability . It is a potent oxidizer (UN 2015, Hazard Class 5.1) and reacts violently with metals, reducing agents, and organic compounds . The term "Hydrogenperoxide(1-)" likely refers to the hydroperoxide anion (HO₂⁻), formed by deprotonation of H₂O₂. While H₂O₂ is well-documented in industrial, biological, and environmental contexts , HO₂⁻ is less studied but plays critical roles in redox chemistry and biological systems, such as in superoxide dismutase-mediated pathways .
Scientific Research Applications
Healthcare Applications
Disinfection and Sterilization
Hydrogen peroxide is widely utilized as a high-level disinfectant in healthcare settings. Its strong oxidizing properties enable it to effectively kill bacteria, viruses, and fungi. Studies have shown that hydrogen peroxide vapor significantly reduces healthcare-associated infections (HCAIs), including those caused by antibiotic-resistant organisms like Methicillin-resistant Staphylococcus aureus (MRSA) .
Case Study: Infection Control in Hospitals
A study conducted by University College London Hospitals demonstrated that the use of hydrogen peroxide vapor, combined with blue light treatments, resulted in a marked reduction in infection rates in surgical wards. The implementation of this protocol improved hygiene standards and patient outcomes .
Therapeutic Uses
Emerging research suggests that hydrogen peroxide may play a role in cancer therapy by inducing apoptosis in cancer cells through its prooxidant properties. This potential therapeutic application is still under investigation but shows promise for future treatment modalities .
Agricultural and Food Processing Applications
Pest Control and Food Safety
In agriculture, hydrogen peroxide is employed to sanitize equipment and surfaces, ensuring the safety of food products. It effectively eliminates pathogens from fresh produce, thereby reducing the risk of foodborne illnesses .
Water Treatment
Hydrogen peroxide is also utilized in water treatment processes to purify drinking water and disinfect swimming pools. Its decomposition into harmless byproducts (water and oxygen) makes it an environmentally friendly alternative to traditional chemical disinfectants .
Environmental Applications
Pollution Control
Hydrogen peroxide is used in environmental remediation efforts to treat contaminated soil and groundwater. Its oxidative properties enable it to break down organic pollutants efficiently .
Safety Assessments
Despite its numerous applications, safety concerns regarding hydrogen peroxide's toxicity must be addressed. Acute toxicity studies indicate that hydrogen peroxide can cause local irritation and systemic effects at high concentrations. For instance, inhalation exposure to high concentrations has resulted in pulmonary edema in animal studies . Therefore, appropriate handling and concentration control are critical when using hydrogen peroxide.
Table 1: Efficacy of Hydrogen Peroxide Concentrations
Concentration (%) | Application | Efficacy Level |
---|---|---|
3% | Surface Disinfection | Effective against most bacteria |
7-30% | Sporicidal Activity | High efficacy against spores |
35% | Industrial Sterilization | Broad-spectrum antimicrobial |
Table 2: Safety Profile of Hydrogen Peroxide
Exposure Route | Observed Effects | LD50 (mg/kg) |
---|---|---|
Oral (70% solution) | Severe gastrointestinal damage | 500-1270 |
Dermal (90% solution) | Skin irritation | Varies |
Inhalation | Pulmonary edema | No deaths reported |
Q & A
Basic Research Questions
Q. How can peroxides in stored Hydrogenperoxide(1-) samples be reliably detected and quantified in laboratory settings?
- Methodological Answer : Use peroxide test strips (Method A) for routine detection of inorganic/organic peroxides in simple ethers like diethyl ether, ensuring the chemical is not expired . For broader applicability, employ the iodide test (Method B), which detects peroxides via iodine release in acidic conditions. Calibrate results against known peroxide concentrations and validate using structural risk factors (e.g., susceptibility of C-H bonds to radical polymerization) outlined in Table 1 of Kelly’s guidelines . Note that test strips may fail for complex peroxosolvates; confirm with spectroscopic methods (e.g., FTIR) .
Q. What stabilization techniques are effective for inhibiting Hydrogenperoxide(1-) decomposition during experiments?
- Methodological Answer : Add inhibitors like acetanilide (0.1–1.0 wt%) to suppress trace-metal-catalyzed decomposition . Store solutions in dark, vented containers at pH 4–6 and ≤25°C. Pre-treat glassware with nitric acid to remove metal residues. Monitor stability via periodic peroxide testing and calorimetric analysis of heat release during decomposition .
Q. What protocols ensure safe handling and storage of Hydrogenperoxide(1-) in academic labs?
- Methodological Answer : Label containers with dates (received, opened, discard-by) and peroxide test results . Store separately from reducing agents, metals (e.g., Cu, Fe), and combustibles. Use secondary containment for concentrated solutions (>30%). Train personnel in spill response (neutralize with sodium sulfite) and emergency first aid for exposures .
Advanced Research Questions
Q. How can researchers design experiments to study Hydrogenperoxide(1-) coordination in peroxosolvates with metal sulfates?
- Methodological Answer : Synthesize peroxosolvates by crystallizing Mg/Ca/Zn sulfates in H₂O₂-rich solutions under controlled humidity . Characterize coordination via X-ray diffraction and Raman spectroscopy to confirm O–O bond retention. Compare decomposition kinetics (O₂ release) with non-peroxosolvate analogs using manometric methods .
Q. What methodologies resolve contradictions in free radical mechanisms during H₂O₂-mediated polysaccharide degradation?
- Methodological Answer : Conduct systematic reviews of ROS pathways using databases (Web of Science, PubMed) with keywords: polysaccharide degradation, H₂O₂ free radicals . Validate mechanisms via electron paramagnetic resonance (EPR) to detect hydroxyl radicals (•OH) and quantify oxidative cleavage using size-exclusion chromatography. Control variables like pH, transition metals, and H₂O₂ concentration to isolate radical contributions .
Q. How can enzymatic effects on Hydrogenperoxide(1-) reactivity be systematically evaluated in biological models?
- Methodological Answer : Treat cell cultures (e.g., chondrocytes) with 0.1 mmol/L H₂O₂ and measure apoptosis (MTT assay), lactate dehydrogenase (LDH), and malondialdehyde (MDA) levels . Pre-treat with antioxidants (e.g., salvia miltiorrhiza) to assess ROS scavenging. Use ANOVA to analyze dose-dependent protection and confocal microscopy for real-time ROS imaging .
Q. What experimental approaches quantify the thermodynamic stability of Hydrogenperoxide(1-) under varying conditions?
- Methodological Answer : Measure decomposition enthalpy (ΔH) via adiabatic calorimetry, comparing inhibited vs. uninhibited H₂O₂ . Correlate ΔH with impurity levels (e.g., metal ions) using ICP-MS. Model stability trends using Arrhenius plots to predict shelf life under accelerated aging conditions (e.g., 40°C) .
Q. How do structural factors influence peroxide formation risks in Hydrogenperoxide(1-) derivatives, and how can these be prioritized in experimental design?
- Methodological Answer : Rank peroxide-forming propensity using computational tools (e.g., bond dissociation energy calculations for C-H bonds) and experimental data from Kelly’s structural analysis . Test high-risk compounds (e.g., ethers, aldehydes) monthly with iodide titration and track peroxide accumulation vs. storage duration .
Q. Data Contradiction Analysis
Q. How can researchers address discrepancies in peroxide detection results across different analytical methods?
- Methodological Answer : Cross-validate test strips (Method A) with iodometric titration (Method B) for the same sample batch . Investigate interference from stabilizers (e.g., acetanilide) or matrix effects (e.g., organic solvents) using spiked recovery experiments. Publish method-specific LOD/LOQ values to guide protocol selection .
Q. What strategies reconcile conflicting data on H₂O₂’s role in ROS-mediated cellular damage vs. signaling?
- Methodological Answer : Perform dual-concentration studies: low-dose (1–10 µM) for signaling (e.g., NF-κB activation) and high-dose (>100 µM) for oxidative stress (e.g., lipid peroxidation) . Use siRNA knockdown of antioxidant enzymes (e.g., catalase) to clarify threshold effects. Meta-analyze published dose-response curves to identify consensus mechanisms .
Comparison with Similar Compounds
Comparison with Structurally Similar Compounds
Peroxide Family: H₂O₂ vs. Sodium Peroxide (Na₂O₂)
- Chemical Stability: H₂O₂ decomposes spontaneously into H₂O and O₂, accelerated by catalysts (e.g., Fe²⁺, MnO₂) . Na₂O₂, a solid ionic peroxide, is more stable but reacts explosively with water, releasing H₂O₂ and NaOH . Decomposition Rates:
- H₂O₂ (30%) decomposes at 0.5 nmol/10⁴ cells/hour in tumor cells, driven by flavoproteins .
Na₂O₂ decomposition requires strong acids or moisture, generating heat (>300°C) .
Applications :
Reactive Oxygen Species (ROS): HO₂⁻ vs. Superoxide (O₂⁻)
Reactivity :
Biological Roles :
Comparison with Other Oxidizers
Ozone (O₃)
- Environmental Impact :
Sodium Hypochlorite (NaOCl)
- Disinfection Efficiency: NaOCl (bleach) has broader antimicrobial activity but generates carcinogenic chlorinated byproducts . H₂O₂ is preferred in food and medical industries for residue-free sterilization .
Data Tables
Table 1: Key Properties of H₂O₂ and Comparable Compounds
Table 2: Decomposition Rates of H₂O₂ Under Different Conditions
Catalyst | Rate (mol/L·s) | Temperature (°C) | pH | Reference |
---|---|---|---|---|
None (3% solution) | 1.2 × 10⁻⁶ | 25 | 7.0 | |
MnO₂ | 4.5 × 10⁻³ | 25 | 7.0 | |
Fe²⁺ | 2.8 × 10⁻² | 37 | 5.0 | |
Catalase (enzyme) | 1.1 × 10⁴ | 37 | 7.4 |
Research Findings
- Biological Production : Human tumor cells generate H₂O₂ at rates up to 0.5 nmol/10⁴ cells/hour, driven by flavoproteins and divalent cations .
- Electrochemical Synthesis: Quinone-modified electrodes enable efficient H₂O₂ production via oxygen reduction, offering advantages over traditional anthraquinone processes .
- Environmental Sinks : Marsh sediments exhibit unexplained H₂O₂ consumption, suggesting uncharacterized redox pathways or microbial activity .
Properties
InChI |
InChI=1S/H2O2/c1-2/h1-2H/p-1 | |
---|---|---|
Details | Computed by InChI 1.0.6 (PubChem release 2021.10.14) | |
Source | PubChem | |
URL | https://pubchem.ncbi.nlm.nih.gov | |
Description | Data deposited in or computed by PubChem | |
InChI Key |
MHAJPDPJQMAIIY-UHFFFAOYSA-M | |
Details | Computed by InChI 1.0.6 (PubChem release 2021.10.14) | |
Source | PubChem | |
URL | https://pubchem.ncbi.nlm.nih.gov | |
Description | Data deposited in or computed by PubChem | |
Canonical SMILES |
O[O-] | |
Details | Computed by OEChem 2.3.0 (PubChem release 2021.10.14) | |
Source | PubChem | |
URL | https://pubchem.ncbi.nlm.nih.gov | |
Description | Data deposited in or computed by PubChem | |
Molecular Formula |
HO2- | |
Details | Computed by PubChem 2.2 (PubChem release 2021.10.14) | |
Source | PubChem | |
URL | https://pubchem.ncbi.nlm.nih.gov | |
Description | Data deposited in or computed by PubChem | |
DSSTOX Substance ID |
DTXSID30894777 | |
Record name | Perhydroxyl radical | |
Source | EPA DSSTox | |
URL | https://comptox.epa.gov/dashboard/DTXSID30894777 | |
Description | DSSTox provides a high quality public chemistry resource for supporting improved predictive toxicology. | |
Molecular Weight |
33.007 g/mol | |
Details | Computed by PubChem 2.2 (PubChem release 2021.10.14) | |
Source | PubChem | |
URL | https://pubchem.ncbi.nlm.nih.gov | |
Description | Data deposited in or computed by PubChem | |
CAS No. |
14691-59-9, 3170-83-0 | |
Record name | Hydroperoxide(1-) | |
Source | CAS Common Chemistry | |
URL | https://commonchemistry.cas.org/detail?cas_rn=14691-59-9 | |
Description | CAS Common Chemistry is an open community resource for accessing chemical information. Nearly 500,000 chemical substances from CAS REGISTRY cover areas of community interest, including common and frequently regulated chemicals, and those relevant to high school and undergraduate chemistry classes. This chemical information, curated by our expert scientists, is provided in alignment with our mission as a division of the American Chemical Society. | |
Explanation | The data from CAS Common Chemistry is provided under a CC-BY-NC 4.0 license, unless otherwise stated. | |
Record name | Perhydroxyl radical | |
Source | ChemIDplus | |
URL | https://pubchem.ncbi.nlm.nih.gov/substance/?source=chemidplus&sourceid=0003170830 | |
Description | ChemIDplus is a free, web search system that provides access to the structure and nomenclature authority files used for the identification of chemical substances cited in National Library of Medicine (NLM) databases, including the TOXNET system. | |
Record name | Peroxide (HO21-) | |
Source | ChemIDplus | |
URL | https://pubchem.ncbi.nlm.nih.gov/substance/?source=chemidplus&sourceid=0014691599 | |
Description | ChemIDplus is a free, web search system that provides access to the structure and nomenclature authority files used for the identification of chemical substances cited in National Library of Medicine (NLM) databases, including the TOXNET system. | |
Record name | Perhydroxyl radical | |
Source | EPA DSSTox | |
URL | https://comptox.epa.gov/dashboard/DTXSID30894777 | |
Description | DSSTox provides a high quality public chemistry resource for supporting improved predictive toxicology. | |
Synthesis routes and methods I
Procedure details
Synthesis routes and methods II
Procedure details
Synthesis routes and methods III
Procedure details
Retrosynthesis Analysis
AI-Powered Synthesis Planning: Our tool employs the Template_relevance Pistachio, Template_relevance Bkms_metabolic, Template_relevance Pistachio_ringbreaker, Template_relevance Reaxys, Template_relevance Reaxys_biocatalysis model, leveraging a vast database of chemical reactions to predict feasible synthetic routes.
One-Step Synthesis Focus: Specifically designed for one-step synthesis, it provides concise and direct routes for your target compounds, streamlining the synthesis process.
Accurate Predictions: Utilizing the extensive PISTACHIO, BKMS_METABOLIC, PISTACHIO_RINGBREAKER, REAXYS, REAXYS_BIOCATALYSIS database, our tool offers high-accuracy predictions, reflecting the latest in chemical research and data.
Strategy Settings
Precursor scoring | Relevance Heuristic |
---|---|
Min. plausibility | 0.01 |
Model | Template_relevance |
Template Set | Pistachio/Bkms_metabolic/Pistachio_ringbreaker/Reaxys/Reaxys_biocatalysis |
Top-N result to add to graph | 6 |
Feasible Synthetic Routes
Disclaimer and Information on In-Vitro Research Products
Please be aware that all articles and product information presented on BenchChem are intended solely for informational purposes. The products available for purchase on BenchChem are specifically designed for in-vitro studies, which are conducted outside of living organisms. In-vitro studies, derived from the Latin term "in glass," involve experiments performed in controlled laboratory settings using cells or tissues. It is important to note that these products are not categorized as medicines or drugs, and they have not received approval from the FDA for the prevention, treatment, or cure of any medical condition, ailment, or disease. We must emphasize that any form of bodily introduction of these products into humans or animals is strictly prohibited by law. It is essential to adhere to these guidelines to ensure compliance with legal and ethical standards in research and experimentation.