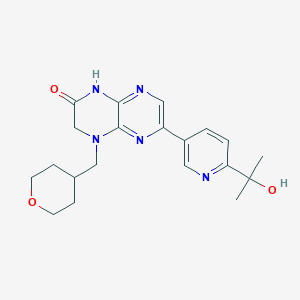
CC214-2
Overview
Description
CC214-2 is an orally active and selective inhibitor of the mammalian target of rapamycin (mTOR) kinase. It targets both mTOR complex 1 (mTORC1) and mTOR complex 2 (mTORC2), making it a potent dual inhibitor. This compound has shown promise in inducing autophagy, a process that can be leveraged for host-directed therapy in tuberculosis. Additionally, this compound exhibits synergistic bactericidal and sterilizing activity against tuberculosis and has demonstrated efficacy in reducing the growth of glioblastomas .
Preparation Methods
Synthetic Routes and Reaction Conditions
The synthesis of CC214-2 involves a series of chemical reactions starting from commercially available precursors. The key steps include:
Formation of the core structure: This involves the construction of a pyrazino[2,3-b]pyrazine-2(1H)-one core through a series of condensation and cyclization reactions.
Substitution reactions: Introduction of various substituents at specific positions on the core structure to enhance the compound’s potency and selectivity.
Purification: The final product is purified using techniques such as recrystallization or chromatography to achieve high purity.
Industrial Production Methods
In an industrial setting, the production of this compound would involve scaling up the synthetic routes mentioned above. This includes optimizing reaction conditions, such as temperature, pressure, and solvent choice, to maximize yield and minimize impurities. Continuous flow chemistry and automated synthesis platforms may be employed to enhance efficiency and reproducibility .
Chemical Reactions Analysis
Types of Reactions
CC214-2 undergoes several types of chemical reactions, including:
Oxidation: The compound can be oxidized under specific conditions to form oxidized derivatives.
Reduction: Reduction reactions can be used to modify certain functional groups within the molecule.
Substitution: Various substitution reactions can be performed to introduce different substituents, enhancing the compound’s properties.
Common Reagents and Conditions
Oxidation: Common oxidizing agents such as hydrogen peroxide or potassium permanganate can be used.
Reduction: Reducing agents like sodium borohydride or lithium aluminum hydride are typically employed.
Substitution: Reagents such as alkyl halides or acyl chlorides are used in substitution reactions.
Major Products Formed
The major products formed from these reactions include various derivatives of this compound with modified functional groups, which can be further studied for their biological activity and therapeutic potential .
Scientific Research Applications
CC214-2 has a wide range of scientific research applications, including:
Chemistry: Used as a tool compound to study mTOR signaling pathways and their role in various cellular processes.
Biology: Investigated for its ability to induce autophagy and its potential as a host-directed therapy in tuberculosis.
Medicine: Explored for its anti-tumor activity, particularly in glioblastomas and other cancers with hyperactivated mTOR pathways.
Industry: Potential applications in the development of new therapeutic agents targeting mTOR signaling .
Mechanism of Action
CC214-2 exerts its effects by selectively inhibiting the kinase activity of mTORC1 and mTORC2. This inhibition disrupts mTOR signaling, leading to the induction of autophagy and inhibition of cell proliferation. The compound targets specific phosphorylation sites on mTORC1 (pS6) and mTORC2 (pAktS473), effectively blocking their downstream signaling pathways. This mechanism is particularly effective in tumors with hyperactivated mTOR pathways, such as glioblastomas .
Comparison with Similar Compounds
Similar Compounds
CC214-1: Another mTOR kinase inhibitor with similar properties but different pharmacokinetic profiles.
Rapamycin: An allosteric inhibitor of mTOR, which has shown limited efficacy in certain cancers due to incomplete suppression of mTOR signaling.
Everolimus: A derivative of rapamycin with improved pharmacokinetics and broader clinical applications.
Uniqueness of CC214-2
This compound is unique in its ability to potently inhibit both mTORC1 and mTORC2, making it a more comprehensive inhibitor compared to rapamycin and its derivatives. Its dual inhibition mechanism and ability to induce autophagy provide a distinct advantage in treating diseases with hyperactivated mTOR pathways .
Properties
Molecular Formula |
C20H25N5O3 |
---|---|
Molecular Weight |
383.4 g/mol |
IUPAC Name |
2-[6-(2-hydroxypropan-2-yl)pyridin-3-yl]-8-(oxan-4-ylmethyl)-5,7-dihydropyrazino[2,3-b]pyrazin-6-one |
InChI |
InChI=1S/C20H25N5O3/c1-20(2,27)16-4-3-14(9-21-16)15-10-22-18-19(23-15)25(12-17(26)24-18)11-13-5-7-28-8-6-13/h3-4,9-10,13,27H,5-8,11-12H2,1-2H3,(H,22,24,26) |
InChI Key |
UWUPKVZQISLSSA-UHFFFAOYSA-N |
Canonical SMILES |
CC(C)(C1=NC=C(C=C1)C2=CN=C3C(=N2)N(CC(=O)N3)CC4CCOCC4)O |
Origin of Product |
United States |
Synthesis routes and methods I
Procedure details
Synthesis routes and methods II
Procedure details
Disclaimer and Information on In-Vitro Research Products
Please be aware that all articles and product information presented on BenchChem are intended solely for informational purposes. The products available for purchase on BenchChem are specifically designed for in-vitro studies, which are conducted outside of living organisms. In-vitro studies, derived from the Latin term "in glass," involve experiments performed in controlled laboratory settings using cells or tissues. It is important to note that these products are not categorized as medicines or drugs, and they have not received approval from the FDA for the prevention, treatment, or cure of any medical condition, ailment, or disease. We must emphasize that any form of bodily introduction of these products into humans or animals is strictly prohibited by law. It is essential to adhere to these guidelines to ensure compliance with legal and ethical standards in research and experimentation.