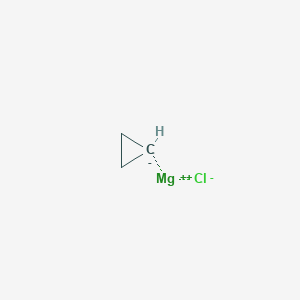
Cyclopropylmagnesium chloride
- Click on QUICK INQUIRY to receive a quote from our team of experts.
- With the quality product at a COMPETITIVE price, you can focus more on your research.
Overview
Description
Cyclopropylmagnesium chloride (C₃H₅MgCl) is a Grignard reagent featuring a cyclopropane ring attached to a magnesium-chloride group. It is widely used in organic synthesis to introduce the cyclopropyl moiety into target molecules, particularly in pharmaceuticals and agrochemicals. The cyclopropyl group is prized for its ring strain, which imparts unique reactivity and conformational rigidity to molecules .
Chemical Reactions Analysis
Preparation via Sulfoxide–Magnesium Exchange
Cyclopropylmagnesium chloride is synthesized through sulfoxide–magnesium exchange using trans-cyclopropyl sulfoxides and iPrMgCl. Key observations include:
-
Reaction Completion : Exchange occurs within 10 minutes at −78°C .
-
Stability : The Grignard intermediate remains stable for ≥2 hours at −78°C, with minimal decomposition at 0°C (<25% yield loss after 2 hours) .
-
Stereochemical Retention : Both E- and Z-cyclopropyl sulfoxides yield products with retained configuration (e.g., cyclopropyl iodides 19a and 20a ) .
Table 1: Stability of this compound Under Varied Conditions
Temperature (°C) | Incubation Time (h) | Yield Retention (%) |
---|---|---|
−78 | 2 | >95 |
0 | 2 | ~75 |
25 | 0.75 | ~50 |
Electrophilic Trapping Reactions
The reagent reacts with diverse electrophiles, enabling functionalization of the cyclopropane ring:
-
Iodination : Trapping with I₂ produces cyclopropyl iodides (trans-diastereomers) in >80% yield .
-
Carbonyl Addition : Reacts with aldehydes/ketones to form alcohols, though competing ester coordination may occur .
-
Epoxide Ring-Opening : Undergoes Sₙ2 attack on epoxides at the least substituted carbon, forming alcohols after acid quenching .
Table 2: Reaction Outcomes with Selected Electrophiles
Electrophile | Product | Yield (%) | Diastereoselectivity |
---|---|---|---|
I₂ | Cyclopropyl iodide | 85–88 | >99% trans |
CH₃CHO | Cyclopropanol | 65–70 | Syn-directed |
Glyoxal derivatives | Tetrahydrofurans | 71–85 | Temperature-dependent |
Stereochemical Integrity and Configurational Stability
-
Retention of Configuration : Reactions with chiral cyclopropanes (e.g., S-(+)-1-bromide) yield Grignard reagents with 15–27% optical purity, confirming partial retention .
-
Halogen Dependence : Configurational stability follows Cl > Br > I, with racemization observed for iodide derivatives .
-
Thermal Sensitivity : Decomposition accelerates above 0°C, leading to reduced yields and stereochemical scrambling .
Coupling and Cycloaddition Reactions
-
Cross-Coupling : Catalyzed by CuI or FeCl₃, it forms quaternary stereocenters via carbometalation of cyclopropenes (e.g., syn-addition to ester-functionalized cyclopropenes) .
-
Diastereodivergent Pathways : Temperature-controlled ring-opening of silylmethylcyclopropanes with SnCl₄ yields cis- or trans-tetrahydrofurans (e.g., 71–85% yield) .
Limitations and Challenges
Q & A
Basic Research Questions
Q. How can the stability of cyclopropylmagnesium chloride in different solvents be systematically evaluated?
this compound is highly air- and moisture-sensitive. To assess solvent stability, prepare solutions in anhydrous tetrahydrofuran (THF), 2-methyltetrahydrofuran (2-MeTHF), or diethyl ether under inert conditions. Monitor reactivity over time using quantitative NMR or titration methods to measure residual Grignard activity. Compare decomposition rates (e.g., via gas evolution or precipitate formation) in solvents with varying donor strengths .
Q. What experimental precautions are critical for handling this compound in nucleophilic additions?
Use rigorous inert-atmosphere techniques (Schlenk lines or gloveboxes) and dry, oxygen-free solvents. Pre-cool reaction vessels to –20°C to –40°C for controlled reactivity. Quench excess reagent with saturated ammonium chloride or methanol, and validate reaction completion via TLC or in situ IR spectroscopy .
Q. How can the concentration of this compound solutions be accurately determined?
Employ titration with a standardized solution of 1,10-phenanthroline-ferrous complex or iodine. Alternatively, use quantitative 1H NMR with a known internal standard (e.g., 1,3,5-trimethoxybenzene) to measure the methylene proton signal of the cyclopropyl group at δ 0.5–1.5 ppm .
Advanced Research Questions
Q. What mechanistic insights explain the divergent reactivity of this compound with α,β-unsaturated carbonyls under varying temperatures?
At low temperatures (–78°C), the reagent preferentially undergoes 1,2-addition due to kinetic control, while higher temperatures (0°C to 25°C) favor 1,4-addition (thermodynamic control). Use DFT calculations to model transition states and validate with 13C NMR kinetic studies. Compare yields of isolated adducts under both conditions .
Q. How do steric and electronic effects influence the coupling efficiency of this compound in cross-coupling reactions?
Steric hindrance from bulky ligands on catalysts (e.g., Pd(PtBu3)2) slows transmetallation but improves selectivity. Electronic effects are probed by substituting the cyclopropyl group with electron-withdrawing/donating groups. Analyze turnover frequencies (TOF) and characterize products via GC-MS or X-ray crystallography .
Q. What strategies resolve contradictions in reported yields for cyclopropane ring-opening reactions mediated by this compound?
Contradictions may arise from trace moisture, solvent polarity, or competing pathways. Replicate experiments under strictly anhydrous conditions and use additives (e.g., LiCl) to modulate reactivity. Perform Hammett analysis to correlate substituent effects with reaction outcomes .
Q. Methodological Considerations
Q. How can side reactions during this compound synthesis be minimized?
Optimize the magnesium activation step by pre-treating with iodine or 1,2-dibromoethane. Use high-purity cyclopropyl chloride and avoid excess halide, which promotes Wurtz coupling. Monitor reaction progress via gas chromatography to detect byproducts like bicyclo[1.1.0]butane .
Q. What analytical techniques are most effective for characterizing this compound adducts?
Combine 1H/13C NMR for structural elucidation, high-resolution mass spectrometry (HRMS) for molecular formula confirmation, and X-ray diffraction for stereochemical assignment. For unstable intermediates, use low-temperature NMR or cryogenic trapping .
Q. Safety and Best Practices
Q. How should accidental exposure to this compound be managed in laboratory settings?
Immediate steps:
- Skin contact: Rinse with 1% acetic acid, followed by water.
- Inhalation: Move to fresh air; administer oxygen if needed. Maintain emergency neutralizing agents (e.g., dry sand, CO2 extinguishers) and train personnel in spill response protocols .
Comparison with Similar Compounds
Reaction Conditions :
- Typically prepared in tetrahydrofuran (THF) at 0°C and quenched with NH₄Cl .
- Stable under inert atmospheres but highly moisture-sensitive.
Comparison with Similar Organomagnesium Compounds
Cyclopropylmagnesium Bromide (C₃H₅MgBr)
Key Differences :
- Reactivity : Bromide-based Grignard reagents are generally more reactive than chloride analogs due to the weaker Mg-Br bond, facilitating faster nucleophilic attacks .
- Solubility : Often prepared in 2-methyltetrahydrofuran (2-MeTHF), a greener solvent, whereas the chloride is primarily used in THF .
- Commercial Availability : Cyclopropylmagnesium bromide is more commercially accessible (e.g., 25 mL, 0.5 M in THF) , while the chloride is less commonly listed, suggesting in situ preparation .
Phenylmagnesium Chloride (C₆H₅MgCl)
Reactivity :
- Higher nucleophilicity toward aromatic systems compared to cyclopropyl derivatives.
- Less strained structure reduces ring-opening side reactions.
Isopropylmagnesium Chloride ((CH₃)₂CHMgCl)
Reactivity :
- Bulkier isopropyl group reduces steric hindrance compared to cyclopropyl, favoring reactions with less sterically demanding electrophiles.
Data Table: Comparative Properties of Selected Grignard Reagents
Reactivity and Stability Considerations
- Halide Influence : Chloride-based reagents (e.g., C₃H₅MgCl) are less reactive but more stable than bromide analogs, enabling better control in stereoselective reactions .
- Ring Strain : The cyclopropyl group’s 60° bond angles promote ring-opening under acidic conditions, a unique feature absent in linear or branched alkyl Grignard reagents .
- Solvent Effects : 2-MeTHF improves the sustainability of bromide reagents, while THF remains standard for chlorides .
Industrial and Research Trends
Properties
Molecular Formula |
C3H5ClMg |
---|---|
Molecular Weight |
100.83 g/mol |
IUPAC Name |
magnesium;cyclopropane;chloride |
InChI |
InChI=1S/C3H5.ClH.Mg/c1-2-3-1;;/h1H,2-3H2;1H;/q-1;;+2/p-1 |
InChI Key |
AAWGSOZCJNFZAG-UHFFFAOYSA-M |
Canonical SMILES |
C1C[CH-]1.[Mg+2].[Cl-] |
Origin of Product |
United States |
Disclaimer and Information on In-Vitro Research Products
Please be aware that all articles and product information presented on BenchChem are intended solely for informational purposes. The products available for purchase on BenchChem are specifically designed for in-vitro studies, which are conducted outside of living organisms. In-vitro studies, derived from the Latin term "in glass," involve experiments performed in controlled laboratory settings using cells or tissues. It is important to note that these products are not categorized as medicines or drugs, and they have not received approval from the FDA for the prevention, treatment, or cure of any medical condition, ailment, or disease. We must emphasize that any form of bodily introduction of these products into humans or animals is strictly prohibited by law. It is essential to adhere to these guidelines to ensure compliance with legal and ethical standards in research and experimentation.