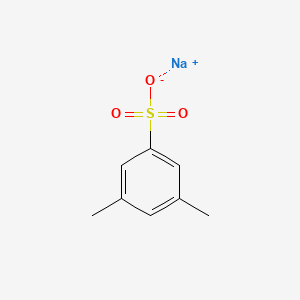
Sodium 5-m-xylene sulfonate
- Click on QUICK INQUIRY to receive a quote from our team of experts.
- With the quality product at a COMPETITIVE price, you can focus more on your research.
Overview
Description
Sodium 5-m-xylene sulfonate is an organic compound with the molecular formula C8H9NaO3S. It is a sodium salt of xylene sulfonic acid and is commonly used as a hydrotrope, which helps to increase the solubility of hydrophobic compounds in water. This compound is particularly valued in various industrial applications due to its ability to enhance the performance of cleaning agents and other formulations.
Preparation Methods
Synthetic Routes and Reaction Conditions: Sodium 5-m-xylene sulfonate can be synthesized through the sulfonation of m-xylene using sulfur trioxide or oleum, followed by neutralization with sodium hydroxide. The reaction typically involves the following steps:
Sulfonation: m-Xylene is reacted with sulfur trioxide or oleum to form m-xylene sulfonic acid.
Neutralization: The resulting m-xylene sulfonic acid is then neutralized with sodium hydroxide to produce this compound.
Industrial Production Methods: In industrial settings, the production of this compound often involves continuous processes using reactors such as falling film reactors. This method ensures efficient sulfonation and neutralization, resulting in high yields and purity of the final product .
Chemical Reactions Analysis
Types of Reactions: Sodium 5-m-xylene sulfonate primarily undergoes substitution reactions due to the presence of the sulfonate group. It can also participate in oxidation and reduction reactions under specific conditions.
Common Reagents and Conditions:
Substitution Reactions: this compound can react with nucleophiles such as amines and alcohols to form sulfonamide and sulfonate ester derivatives, respectively.
Oxidation Reactions: Under strong oxidizing conditions, the aromatic ring of this compound can be oxidized to form sulfone derivatives.
Reduction Reactions: The sulfonate group can be reduced to a sulfinate group using reducing agents like sodium borohydride.
Major Products Formed:
Sulfonamide Derivatives: Formed by reacting with amines.
Sulfonate Ester Derivatives: Formed by reacting with alcohols.
Sulfone Derivatives: Formed under oxidizing conditions.
Scientific Research Applications
Sodium 5-m-xylene sulfonate has a wide range of applications in scientific research and industry:
Chemistry: Used as a hydrotrope to enhance the solubility of hydrophobic compounds in aqueous solutions, facilitating various chemical reactions and processes.
Biology: Employed in biochemical assays and experiments to improve the solubility of biological molecules.
Medicine: Investigated for its potential use in drug formulations to enhance the solubility and bioavailability of poorly soluble drugs.
Mechanism of Action
The primary mechanism by which sodium 5-m-xylene sulfonate exerts its effects is through its hydrotropic properties. As a hydrotrope, it increases the solubility of hydrophobic compounds in water by disrupting the structure of water and reducing the surface tension. This allows for better interaction between water and hydrophobic molecules, enhancing their solubility and stability in aqueous solutions .
Comparison with Similar Compounds
Sodium 5-m-xylene sulfonate can be compared with other similar hydrotropic compounds such as:
- Sodium p-xylene sulfonate
- Sodium benzene sulfonate
- Sodium cumene sulfonate
- Sodium p-toluene sulfonate
Uniqueness: this compound is unique due to its specific molecular structure, which provides distinct hydrotropic properties. It is particularly effective in increasing the solubility of certain hydrophobic compounds compared to other hydrotropes .
Properties
Molecular Formula |
C8H9NaO3S |
---|---|
Molecular Weight |
208.21 g/mol |
IUPAC Name |
sodium;3,5-dimethylbenzenesulfonate |
InChI |
InChI=1S/C8H10O3S.Na/c1-6-3-7(2)5-8(4-6)12(9,10)11;/h3-5H,1-2H3,(H,9,10,11);/q;+1/p-1 |
InChI Key |
RIQKQQLKIBMABS-UHFFFAOYSA-M |
Canonical SMILES |
CC1=CC(=CC(=C1)S(=O)(=O)[O-])C.[Na+] |
Origin of Product |
United States |
Disclaimer and Information on In-Vitro Research Products
Please be aware that all articles and product information presented on BenchChem are intended solely for informational purposes. The products available for purchase on BenchChem are specifically designed for in-vitro studies, which are conducted outside of living organisms. In-vitro studies, derived from the Latin term "in glass," involve experiments performed in controlled laboratory settings using cells or tissues. It is important to note that these products are not categorized as medicines or drugs, and they have not received approval from the FDA for the prevention, treatment, or cure of any medical condition, ailment, or disease. We must emphasize that any form of bodily introduction of these products into humans or animals is strictly prohibited by law. It is essential to adhere to these guidelines to ensure compliance with legal and ethical standards in research and experimentation.