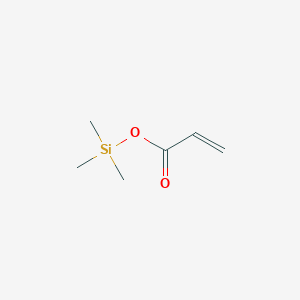
Trimethylsilyl acrylate
Overview
Description
Trimethylsilyl acrylate (TMSA), with the chemical formula C₆H₁₂O₂Si and CAS number 13688-55-6, is a silylated ester of acrylic acid. It is structurally characterized by a trimethylsilyl (-Si(CH₃)₃) group attached to the oxygen of the acrylate moiety. This modification enhances its solubility in non-polar solvents and reduces its polarity, making it valuable in polymer chemistry, surface modification, and organic synthesis . TMSA is frequently utilized as a protecting group for hydroxyl functionalities or as a monomer in controlled radical polymerization (e.g., ATRP, SET-LRP) to introduce hydrophobic or functional side chains .
Preparation Methods
Synthetic Routes and Reaction Conditions: Gallotannins can be synthesized through the esterification of gallic acid with glucose. The reaction typically involves the use of benzyl-protected gallic acid chloride as the substrate . The reaction conditions that lead to high anomeric selectivity for the synthesis of gallotannins include the use of acetonitrile as a solvent .
Industrial Production Methods: Industrially, gallotannins are extracted from plant sources such as gall nuts, sumac, and tara pods. The extraction process involves the use of solvents like water or alcohol to extract the tannins from the plant material. The extract is then purified to obtain gallotannins .
Chemical Reactions Analysis
Types of Reactions: Gallotannins undergo various chemical reactions, including:
Oxidation: Gallotannins can be oxidized to form quinones.
Hydrolysis: They can be hydrolyzed to yield gallic acid and glucose.
Complexation: Gallotannins can form complexes with proteins and metal ions.
Common Reagents and Conditions:
Oxidation: Common oxidizing agents include potassium permanganate and hydrogen peroxide.
Hydrolysis: Acidic or basic conditions can be used for hydrolysis.
Complexation: Gallotannins readily form complexes under neutral or slightly acidic conditions.
Major Products:
Oxidation: Quinones.
Hydrolysis: Gallic acid and glucose.
Complexation: Protein-tannin complexes and metal-tannin complexes.
Scientific Research Applications
Polymer Chemistry
TMSA is widely used in polymer synthesis due to its ability to undergo radical polymerization. The incorporation of the trimethylsilyl group enhances the hydrophobicity and adhesion properties of the resulting polymers, making them suitable for various applications.
- Radical Polymerization : TMSA can be polymerized using radical initiators, leading to the formation of stable polymers. Its low viscosity during polymerization makes it advantageous for industrial applications compared to other acrylates.
- Photo-Induced Polymerization : Recent studies have explored TMSA's role in photo-induced radical polymerization, where it acts as a monomer that can be activated under UV light, leading to efficient polymer formation .
Polymerization Type | Characteristics | Applications |
---|---|---|
Radical Polymerization | Stable polymers with enhanced properties | Coatings, adhesives |
Photo-Induced Polymerization | High reactivity under UV light | Photocurable materials |
Organic Synthesis
TMSA serves as a valuable reagent in organic synthesis. Its silyl group can facilitate various chemical transformations:
- Hydrosilylation Reactions : TMSA can participate in hydrosilylation reactions, allowing for the introduction of silyl groups into organic molecules. This is particularly useful in modifying functional groups to enhance their stability and reactivity .
- Synthesis of Functionalized Polymers : The ability of TMSA to form copolymers with other monomers, such as methacrylic acid, has been explored to improve adhesion properties in coatings and films .
Material Science
The incorporation of TMSA into materials science has led to advancements in developing new materials with desirable properties:
- Adhesives and Sealants : Due to its excellent adhesion properties when incorporated into formulations, TMSA-based materials are used in adhesives and sealants that require water resistance and durability.
- Nanocomposites : Research has shown that TMSA can be used to modify nanocomposite materials, enhancing their mechanical properties and thermal stability .
Case Study 1: Photopolymerization of TMSA
In a study focusing on photopolymerization processes, TMSA was utilized as a monomer in UV-curable coatings. The results indicated that TMSA significantly improved the mechanical properties of the cured films while maintaining transparency and flexibility. This application highlights the potential for TMSA in developing advanced coatings for various industrial applications .
Case Study 2: Copolymer Formation
Another investigation explored the copolymerization of TMSA with methacrylic acid. The resulting copolymers exhibited enhanced adhesion between layers in resist films used in lithography processes. This application demonstrates TMSA's utility in improving performance characteristics in microfabrication technologies .
Mechanism of Action
Gallotannins exert their effects through various mechanisms:
Antioxidant Activity: They scavenge free radicals and reduce oxidative stress.
Enzyme Inhibition: Gallotannins inhibit enzymes such as lipoxygenase and cyclooxygenase, which are involved in inflammatory processes.
Protein Binding: They form complexes with proteins, which can inhibit microbial growth and enzyme activity.
Molecular Targets and Pathways:
JAK/STAT Pathway: Gallotannins modulate this pathway, which is involved in cell proliferation and immune responses.
RAS/RAF/mTOR Pathway: They influence this pathway, which is crucial for cell growth and survival.
Comparison with Similar Compounds
Physical and Chemical Properties
Table 1: Key Properties of TMSA and Related Silylated Acrylates
Compound | Molecular Formula | Molecular Weight (g/mol) | CAS Number | Key Features |
---|---|---|---|---|
Trimethylsilyl acrylate | C₆H₁₂O₂Si | 144.24 | 13688-55-6 | Hydrophobic, enhances solubility |
Trimethylsilyl acetate | C₅H₁₂O₂Si | 132.23 | 13411-48-8 | Simpler ester, volatile |
TMS-T-BTD* | C₁₇H₂₂N₂S₂Si₂ | 386.66 | - | Higher solubility in hexane |
TMSPA** | C₉H₁₄O₂Si | 198.29 | - | Propargyl group for click chemistry |
TMS-T-BTD: 4,7-Di-2-thienyl-2,1,3-benzothiadiazole with terminal trimethylsilyl substituents .
*TMSPA: Trimethylsilyl propargyl acrylate .
Key Observations :
- Solubility: The trimethylsilyl group significantly improves solubility in non-polar solvents. For example, TMS-T-BTD exhibits 1.5 mg/g solubility in hexane, double that of its non-silylated counterpart (0.75 mg/g) .
- Thermal Stability : Silylated compounds often show moderate thermal stability. TMS-T-BTD has a decomposition temperature (T₅%) of 386°C , slightly lower than the unmodified T-BTD (400°C) due to the labile Si-O bond .
Table 2: Reactivity Comparison in Polymer Chemistry
Key Observations :
- TMSA’s trimethylsilyl group can be selectively removed under acidic or fluoride conditions, enabling post-polymerization functionalization .
- TMSPA integrates both acrylate and alkyne functionalities, allowing orthogonal reactivity in multi-step syntheses .
Functional Performance in Material Science
Table 3: Performance in Polymer Networks
Compound | Role in Polymer Networks | Outcome |
---|---|---|
This compound | Hydrophobic monomer | Enhances water resistance in APCNs* |
TMS-HEA** | Crosslinking agent | Improves mechanical stability in APCNs |
APCNs: Amphiphilic polymer conetworks .
*TMS-HEA: Trimethylsilyl hydroxyethyl acrylate .
Key Observations :
- TMSA-based polymers exhibit enhanced solvation and phase separation in nanostructured materials, critical for applications like wearable sensors .
Biological Activity
Trimethylsilyl acrylate (TMSA) is an organosilicon compound characterized by a trimethylsilyl group attached to an acrylate moiety, with the chemical formula C₆H₁₄O₂Si and a molecular weight of approximately 142.26 g/mol. This compound is recognized for its unique reactivity, particularly in organic synthesis and polymer chemistry. Its biological activity has been explored in various contexts, including its potential toxicity and interactions with biological systems.
TMSA is typically synthesized through the reaction of acrylic acid with trimethylsilyl chloride. The resulting product is a colorless to light yellow liquid that is soluble in organic solvents. The presence of the silyl group enhances the compound's reactivity, making it suitable for polymerization reactions and other synthetic applications.
Biological Activity Overview
The biological activity of TMSA can be categorized into several key areas:
- Toxicity : Studies have indicated that acrylates, including TMSA, exhibit hemolytic activity, which refers to their ability to lyse red blood cells. The toxicity is often linked to the compound's reactivity with cellular membranes, leading to membrane disruption . Quantitative structure-activity relationship (QSAR) models have been developed to predict the toxicity of acrylates based on their chemical structure and lipophilicity .
- Polymerization and Biocompatibility : TMSA is utilized in the synthesis of polymers that can be engineered for biocompatibility. For instance, its polymerization can yield materials suitable for biomedical applications, such as drug delivery systems and tissue engineering scaffolds. The incorporation of TMSA into polymer matrices can enhance mechanical properties while maintaining biocompatibility .
- Reactivity with Biological Molecules : The reactivity of TMSA towards nucleophiles, such as glutathione (GSH), has been studied to understand its potential effects on biological systems. The unsaturated β-carbon in acrylates is a primary site for nucleophilic attack, leading to various biological interactions that may result in cytotoxic effects .
Case Study 1: Hemolytic Activity
A study investigating the hemolytic activity of various acrylates found that TMSA exhibited significant hemolytic properties when tested against human erythrocytes. The mechanism was attributed to membrane-mediated interactions where TMSA disrupts lipid bilayers, leading to cell lysis. The half-maximal effective concentration (EC50) was determined through dose-response curves, illustrating a clear correlation between concentration and hemolytic effect .
Compound | EC50 (mM) | Mechanism |
---|---|---|
This compound | 0.5 | Membrane disruption |
Other Acrylates | Varies | Varies |
Case Study 2: Polymer Applications
In another study, TMSA was incorporated into a polymer matrix for drug delivery applications. The resulting polymers demonstrated controlled release profiles for therapeutic agents, indicating that TMSA can enhance the performance of drug delivery systems by providing a stable yet reactive environment for encapsulation .
Research Findings
Recent research has highlighted several important findings regarding the biological activity of TMSA:
- Toxicity Mechanisms : Investigations into the mechanisms of toxicity have shown that TMSA interacts with cellular components through Michael addition reactions, which can lead to oxidative stress and cellular damage .
- Polymerization Behavior : Studies have demonstrated that TMSA can undergo radical polymerization under specific conditions, yielding polymers with desirable properties for biomedical applications. The presence of oxygen during polymerization was found to significantly affect the reactivity and yield of the resulting polymers .
- Biocompatibility : While TMSA exhibits toxicological concerns at certain concentrations, its derivatives and copolymers have shown promise in biocompatibility studies, suggesting potential applications in medical devices and tissue engineering .
Q & A
Basic Research Questions
Q. What are the standard synthetic routes for preparing trimethylsilyl acrylate in laboratory settings?
this compound is typically synthesized via silylation of acrylic acid or its salts using trimethylsilyl chloride (TMSCI) under anhydrous conditions. A common method involves reacting acrylic acid with TMSCI in the presence of a base (e.g., triethylamine) to neutralize HCl byproducts. The reaction is carried out in an inert atmosphere (e.g., nitrogen) to prevent moisture interference, followed by purification via fractional distillation . Alternative routes may employ transesterification with silylating agents, ensuring protection of reactive hydroxyl groups during polymer synthesis .
Q. What precautions are critical when handling this compound to ensure laboratory safety?
Key precautions include:
- Storage : Keep in a dry, ventilated area at 2–8°C, sealed to prevent moisture ingress .
- Incompatibilities : Avoid contact with strong oxidizers (e.g., peroxides), acids, bases, and amines to prevent exothermic reactions .
- PPE : Use nitrile gloves, lab coats, and safety goggles. Work in a fume hood to minimize inhalation risks .
- First Aid : For skin contact, wash immediately with soap and water; for inhalation, move to fresh air and seek medical attention if irritation persists .
Q. Which analytical techniques are most effective for characterizing this compound and its derivatives?
- GC-MS : Trimethylsilyl (TMS) groups enhance volatility, enabling precise analysis of derivatives. Derivatization with agents like MSTFA ensures complete silylation for accurate peak identification .
- NMR Spectroscopy : H and C NMR confirm structural integrity, with characteristic shifts for TMS groups (e.g., δ 0.1–0.3 ppm for Si-CH) .
- FT-IR : Peaks at ~1250 cm (Si-C) and ~840 cm (Si-O) validate silyl group incorporation .
Advanced Research Questions
Q. How can researchers optimize the efficiency of polymer grafting reactions using this compound?
Surface-initiated single-electron transfer living radical polymerization (SET-LRP) is a robust method for grafting TMS-protected acrylates onto substrates like cellulose nanofibrils (CNFs). Key steps include:
- Deprotection : Hydrolysis of TMS groups post-polymerization to expose alkynes for click chemistry .
- Catalyst Optimization : Use Cu(I) catalysts (e.g., CuBr) with ligands (e.g., PMDETA) to enhance reaction control and minimize side reactions .
- Solvent Selection : Polar aprotic solvents (e.g., DMSO) improve monomer solubility and grafting density .
Q. What strategies resolve contradictions in spectroscopic data when analyzing trimethylsilyl-protected compounds?
Discrepancies in GC-MS or NMR data often arise from incomplete derivatization or residual moisture. Mitigation strategies include:
- Derivatization Validation : Use excess MSTFA (75 μL/sample) at 50°C for 30 minutes to ensure complete silylation .
- Cross-Validation : Combine GC-MS with LC-MS or MALDI-TOF to confirm molecular weights and detect impurities .
- Moisture Control : Conduct reactions in anhydrous solvents and use molecular sieves to stabilize TMS derivatives .
Q. How does the choice of silylating agent influence the reactivity and stability of acrylate monomers in radical polymerization?
Trimethylsilyl groups offer steric protection and hydrolytic stability compared to bulkier silyl agents (e.g., tert-butyldimethylsilyl). This balance enables:
- Controlled Reactivity : TMS acrylates participate in radical polymerization without premature termination, unlike more hindered silyl groups .
- Post-Polymerization Modification : Selective deprotection of TMS groups under mild acidic conditions facilitates functionalization (e.g., fluorophore conjugation) .
- Thermal Stability : TMS derivatives resist degradation during high-temperature processing (e.g., >150°C), critical for automotive coatings .
Properties
IUPAC Name |
trimethylsilyl prop-2-enoate | |
---|---|---|
Source | PubChem | |
URL | https://pubchem.ncbi.nlm.nih.gov | |
Description | Data deposited in or computed by PubChem | |
InChI |
InChI=1S/C6H12O2Si/c1-5-6(7)8-9(2,3)4/h5H,1H2,2-4H3 | |
Source | PubChem | |
URL | https://pubchem.ncbi.nlm.nih.gov | |
Description | Data deposited in or computed by PubChem | |
InChI Key |
OTYBJBJYBGWBHB-UHFFFAOYSA-N | |
Source | PubChem | |
URL | https://pubchem.ncbi.nlm.nih.gov | |
Description | Data deposited in or computed by PubChem | |
Canonical SMILES |
C[Si](C)(C)OC(=O)C=C | |
Source | PubChem | |
URL | https://pubchem.ncbi.nlm.nih.gov | |
Description | Data deposited in or computed by PubChem | |
Molecular Formula |
C6H12O2Si | |
Source | PubChem | |
URL | https://pubchem.ncbi.nlm.nih.gov | |
Description | Data deposited in or computed by PubChem | |
DSSTOX Substance ID |
DTXSID00339226 | |
Record name | Trimethylsilyl acrylate | |
Source | EPA DSSTox | |
URL | https://comptox.epa.gov/dashboard/DTXSID00339226 | |
Description | DSSTox provides a high quality public chemistry resource for supporting improved predictive toxicology. | |
Molecular Weight |
144.24 g/mol | |
Source | PubChem | |
URL | https://pubchem.ncbi.nlm.nih.gov | |
Description | Data deposited in or computed by PubChem | |
CAS No. |
13688-55-6 | |
Record name | Trimethylsilyl acrylate | |
Source | EPA DSSTox | |
URL | https://comptox.epa.gov/dashboard/DTXSID00339226 | |
Description | DSSTox provides a high quality public chemistry resource for supporting improved predictive toxicology. | |
Record name | Trimethylsilyl Acrylate (stabilized with BHT) | |
Source | European Chemicals Agency (ECHA) | |
URL | https://echa.europa.eu/information-on-chemicals | |
Description | The European Chemicals Agency (ECHA) is an agency of the European Union which is the driving force among regulatory authorities in implementing the EU's groundbreaking chemicals legislation for the benefit of human health and the environment as well as for innovation and competitiveness. | |
Explanation | Use of the information, documents and data from the ECHA website is subject to the terms and conditions of this Legal Notice, and subject to other binding limitations provided for under applicable law, the information, documents and data made available on the ECHA website may be reproduced, distributed and/or used, totally or in part, for non-commercial purposes provided that ECHA is acknowledged as the source: "Source: European Chemicals Agency, http://echa.europa.eu/". Such acknowledgement must be included in each copy of the material. ECHA permits and encourages organisations and individuals to create links to the ECHA website under the following cumulative conditions: Links can only be made to webpages that provide a link to the Legal Notice page. | |
Retrosynthesis Analysis
AI-Powered Synthesis Planning: Our tool employs the Template_relevance Pistachio, Template_relevance Bkms_metabolic, Template_relevance Pistachio_ringbreaker, Template_relevance Reaxys, Template_relevance Reaxys_biocatalysis model, leveraging a vast database of chemical reactions to predict feasible synthetic routes.
One-Step Synthesis Focus: Specifically designed for one-step synthesis, it provides concise and direct routes for your target compounds, streamlining the synthesis process.
Accurate Predictions: Utilizing the extensive PISTACHIO, BKMS_METABOLIC, PISTACHIO_RINGBREAKER, REAXYS, REAXYS_BIOCATALYSIS database, our tool offers high-accuracy predictions, reflecting the latest in chemical research and data.
Strategy Settings
Precursor scoring | Relevance Heuristic |
---|---|
Min. plausibility | 0.01 |
Model | Template_relevance |
Template Set | Pistachio/Bkms_metabolic/Pistachio_ringbreaker/Reaxys/Reaxys_biocatalysis |
Top-N result to add to graph | 6 |
Feasible Synthetic Routes
Disclaimer and Information on In-Vitro Research Products
Please be aware that all articles and product information presented on BenchChem are intended solely for informational purposes. The products available for purchase on BenchChem are specifically designed for in-vitro studies, which are conducted outside of living organisms. In-vitro studies, derived from the Latin term "in glass," involve experiments performed in controlled laboratory settings using cells or tissues. It is important to note that these products are not categorized as medicines or drugs, and they have not received approval from the FDA for the prevention, treatment, or cure of any medical condition, ailment, or disease. We must emphasize that any form of bodily introduction of these products into humans or animals is strictly prohibited by law. It is essential to adhere to these guidelines to ensure compliance with legal and ethical standards in research and experimentation.