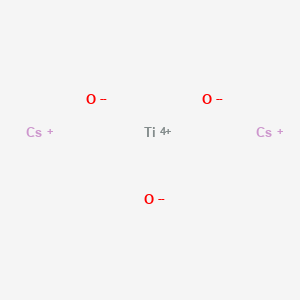
Cesium titanate
Overview
Description
Cesium titanate (Cs₀.₇Ti₁.₈₂₅O₄ or Cs₀.₆₇Ti₁.₈₃O₄) is a layered lepidocrocite-type compound synthesized via solid-state reactions at high temperatures (800°C) using Cs₂CO₃ and TiO₂ precursors . Its structure consists of edge-sharing TiO₆ octahedral layers with cesium ions occupying interlayer spaces. Key properties include:
- Ion-exchange capacity: Interlayer Cs⁺ can be replaced with protons (H⁺) or other cations (e.g., tetrabutylammonium, TBA⁺) to form protonated titanates (H₀.₇Ti₁.₈₂₅O₄·H₂O) or exfoliated titanate nanosheets (TNS) .
- Photocatalytic activity: Undoped this compound absorbs UV light, but Ni-doping introduces visible-light absorption (~500 nm) via localized energy states .
- Adsorption efficiency: Protonated or exfoliated forms exhibit high Cs⁺ adsorption due to increased surface area and active sites .
Preparation Methods
Synthetic Routes and Reaction Conditions: Cesium titanate can be synthesized through solid-state reactions. One common method involves heating a mixture of cesium carbonate (Cs₂CO₃) and anatase titanium dioxide (TiO₂) at a molar ratio of 1:5 at 1000°C for 20 hours . This process yields Cs₂Ti₅O₁₁, a layered this compound.
Industrial Production Methods: Industrial production of this compound typically follows similar solid-state reaction methods, with precise control over temperature and reaction time to ensure the desired phase and purity of the product. Mechanochemical reactions, involving the grinding of reactants in an agate mortar and pestle, are also employed to produce various this compound compounds .
Chemical Reactions Analysis
Types of Reactions: Cesium titanate primarily undergoes ion exchange reactions. It can exchange its cesium ions with other cations such as hydrogen, lithium, and sodium in aqueous solutions . This ion exchange capability is crucial for its applications in removing cesium from contaminated water.
Common Reagents and Conditions: The ion exchange reactions typically occur in aqueous solutions, with the pH and concentration of the solution playing significant roles. For example, the removal rate of cesium ions is about 88% when the pH is 5.00 ± 0.05, with a cesium concentration of 10 ppm and a titanate nanosheet concentration of 0.1 g/L .
Major Products Formed: The major products formed from these ion exchange reactions are the exchanged titanate compounds, such as hydrogen titanate, lithium titanate, and sodium titanate .
Scientific Research Applications
Cesium titanate has a wide range of scientific research applications:
Medicine: Research is ongoing into its potential use in medical applications, particularly in the development of materials for radiation therapy.
Industry: this compound is used in the production of ceramics and as a catalyst in various chemical reactions.
Mechanism of Action
The primary mechanism by which cesium titanate exerts its effects is through ion exchange. The compound has a layered structure with exchangeable cations between the layers. When this compound is introduced into an aqueous solution containing cesium ions, the cesium ions are exchanged with the cations in the titanate, effectively removing the cesium from the solution . This ion exchange process is highly efficient and selective, making this compound an excellent material for cesium ion removal.
Comparison with Similar Compounds
Comparison with Similar Titanate Compounds
Structural and Compositional Differences
Compound | Formula | Structure | Key Features |
---|---|---|---|
Cesium titanate | Cs₀.₇Ti₁.₈₂₅O₄ | Lepidocrocite layered | Interlayer Cs⁺, exfoliable to nanosheets |
Sodium trititanate | Na₂Ti₃O₇ | Layered/tunnel | High selectivity for Cs⁺ in composites |
Monosodium titanate | NaTi₂O₅(OH) | Amorphous/microporous | Contains Na⁺, used in Sr/Cs decontamination |
Crystalline silicotitanate | Na₂Ti₂O₃SiO₄·nH₂O | Microporous framework | High Cs⁺/Sr²⁺ selectivity in ion-exchange |
Titanate nanosheets (TNS) | H₀.₇Ti₁.₈₂₅O₄ | 2D exfoliated layers | Ultrahigh surface area, pH-responsive |
Functional Properties
Adsorption Performance
*Estimated from surface area enhancement in TNS .
Photocatalytic Activity
Ni-doped this compound/TiO₂ composites exhibit 2–3× higher visible-light photocatalytic activity than pure TiO₂ due to enhanced charge separation .
Research Trends and Challenges
- Tailored doping : Transition metals (e.g., Ni, Fe) improve optoelectronic properties but require precise control to avoid phase impurities .
- Scalability : Exfoliation methods for TNS are time-consuming (2 weeks); alternatives like sonication are under exploration .
- Selectivity: Sodium-containing titanates (e.g., monosodium titanate) face limitations in flexible ion-exchange, whereas LHT-9 (Na-free) allows customizable sorption .
Biological Activity
Cesium titanate (CsTiO₃) is a compound that has garnered attention for its potential applications in various fields, including environmental remediation, photocatalysis, and biomedical applications. This article provides a detailed examination of the biological activity associated with this compound, highlighting its interactions with biological systems, cytotoxicity, and potential therapeutic uses.
This compound can be synthesized through various methods, including solid-state reactions and hydrothermal processes. The chemical formula CsTiO₃ indicates that it consists of cesium ions and titanium oxide, forming a perovskite structure that is stable under various conditions. Its unique properties stem from this structure, which allows for ion exchange and photocatalytic activity.
Cytotoxicity Studies
Research has indicated that this compound exhibits varying degrees of cytotoxicity depending on the concentration and exposure duration. For instance, a study showed that this compound nanoparticles had minimal cytotoxic effects on human cell lines at lower concentrations but demonstrated significant cytotoxicity at higher doses. This suggests a dose-dependent relationship where lower concentrations may be safe for cellular interactions while higher concentrations could induce cell death.
Table 1: Cytotoxicity of this compound on Different Cell Lines
Cell Line | Concentration (µg/mL) | Cell Viability (%) | Observations |
---|---|---|---|
HeLa | 10 | 95 | Minimal effect |
HeLa | 100 | 70 | Moderate cytotoxicity |
L929 | 10 | 90 | Minimal effect |
L929 | 100 | 50 | Significant cytotoxicity |
Inflammatory Response
The interaction of this compound with immune cells has been studied to understand its potential as a therapeutic agent. In vitro experiments revealed that this compound could modulate inflammatory responses in monocytes. When exposed to bacterial lipopolysaccharides (LPS), cells treated with this compound exhibited altered cytokine secretion profiles, indicating a potential role in immune modulation.
Case Study: Modulation of Cytokine Secretion
In a study involving THP-1 monocytes, this compound was shown to enhance the secretion of TNF-α when activated by LPS. This suggests that this compound may have immunomodulatory properties that could be harnessed in therapeutic applications.
Photocatalytic Activity and Environmental Applications
This compound is also noted for its photocatalytic properties, particularly in the degradation of organic pollutants. Studies have demonstrated that this compound can effectively degrade dyes and other organic compounds under UV light irradiation. This property is attributed to its ability to generate reactive oxygen species (ROS), which facilitate the breakdown of complex organic molecules.
Table 2: Photocatalytic Efficiency of this compound
Pollutant | Initial Concentration (mg/L) | Degradation Rate (%) | Reaction Time (min) |
---|---|---|---|
Methylene Blue | 10 | 85 | 60 |
Rhodamine B | 10 | 90 | 60 |
Phenol | 5 | 75 | 30 |
Ion Exchange Properties
The ion-exchange capacity of this compound makes it an effective material for removing cesium ions from contaminated water sources. Research indicates that this compound can achieve high removal rates through ion exchange mechanisms, making it a viable candidate for environmental remediation efforts.
Case Study: Removal of Cesium Ions from Water
A study demonstrated that this compound nanosheets could remove up to 88% of Cs⁺ ions from aqueous solutions within 20 minutes, showcasing its potential as an adsorbent for radioactive waste management.
Q & A
Basic Research Questions
Q. What are the standard synthesis methods for cesium titanate, and how do they influence material properties?
this compound is typically synthesized via solid-state reactions or sol-gel processes. Solid-state methods involve high-temperature calcination (e.g., 800–1000°C) of stoichiometric mixtures of cesium carbonate (Cs₂CO₃) and titanium oxide (TiO₂). Sol-gel routes use alkoxide precursors (e.g., titanium isopropoxide) in organic solvents, enabling better stoichiometric control and nanostructuring. The choice of method impacts crystallinity, particle size, and defect density, which are critical for optoelectronic applications .
Q. What characterization techniques are essential for analyzing this compound’s crystal structure and phase purity?
X-ray diffraction (XRD) is primary for phase identification and lattice parameter calculation. High-resolution transmission electron microscopy (HR-TEM) provides nanoscale structural details, while Raman spectroscopy identifies vibrational modes linked to Ti-O bonding. Pair these with energy-dispersive X-ray spectroscopy (EDS) to confirm elemental composition and avoid secondary phases .
Q. How can researchers assess the thermal stability of this compound under varying environmental conditions?
Thermogravimetric analysis (TGA) coupled with differential scanning calorimetry (DSC) quantifies decomposition temperatures and phase transitions. Accelerated aging tests under controlled humidity (e.g., 85% RH at 85°C) and thermal cycling (e.g., −40°C to 120°C) simulate long-term environmental stress, with post-test XRD to detect structural degradation .
Q. What are the key electronic properties of this compound, and how are they measured experimentally?
Bandgap energy is determined via UV-Vis spectroscopy (Tauc plot analysis), while Hall effect measurements quantify carrier concentration and mobility. Photoluminescence (PL) spectroscopy reveals defect-related recombination pathways. For surface properties, X-ray photoelectron spectroscopy (XPS) identifies oxidation states and surface adsorbates .
Advanced Research Questions
Q. How do synthesis parameters (e.g., annealing atmosphere) influence defect density and charge transport in this compound?
Oxygen-deficient atmospheres (e.g., Ar/H₂) during annealing introduce oxygen vacancies, enhancing n-type conductivity but risking structural instability. In contrast, oxidative environments (e.g., O₂) reduce defects but may limit carrier density. Use positron annihilation spectroscopy (PAS) to quantify vacancy concentration and correlate with transport data from impedance spectroscopy .
Q. What strategies can mitigate phase instability in this compound under operational stress (e.g., high voltage or irradiation)?
Doping with aliovalent ions (e.g., Nb⁵+ for Ti⁴+) stabilizes the perovskite lattice by reducing vacancy migration. Encapsulation with inert layers (e.g., Al₂O₃ via atomic layer deposition) minimizes interfacial degradation. In-situ TEM or synchrotron XRD under applied bias/radiation tracks real-time structural evolution .
Q. How can researchers resolve contradictions in reported bandgap values for this compound across studies?
Discrepancies often arise from differences in synthesis routes (e.g., impurity phases) or measurement techniques (e.g., indirect vs. direct bandgap assumptions). Conduct a comparative study using identical synthesis protocols and multiple characterization methods (UV-Vis, ellipsometry, PL) on the same batch. Cross-validate with computational models (DFT) to account for excitonic effects .
Q. What advanced computational methods are used to predict this compound’s optoelectronic behavior?
Density functional theory (DFT) with Hubbard-U corrections models electronic structure and defect thermodynamics. Machine learning (ML) algorithms trained on experimental datasets predict dopant combinations for target properties (e.g., bandgap tuning). Molecular dynamics (MD) simulations assess ion migration barriers under thermal/electrical stress .
Q. How can in-situ spectroscopic techniques elucidate reaction mechanisms during this compound synthesis?
Operando Raman or Fourier-transform infrared (FTIR) spectroscopy tracks intermediate phases during sol-gel processing. High-temperature XRD monitors crystallization kinetics in solid-state reactions. Pair with mass spectrometry (MS) to detect gas-phase byproducts (e.g., CO₂ from Cs₂CO₃ decomposition) .
Q. What methodologies enable reproducible fabrication of this compound thin films for device integration?
Spin-coating or pulsed laser deposition (PLD) ensures uniform thickness. Optimize precursor stoichiometry (e.g., Cs:Ti molar ratios) and post-deposition annealing (ramp rates, dwell time) to minimize pinholes. Use spectroscopic ellipsometry for real-time thickness monitoring and AFM for roughness analysis .
Q. Methodological Guidelines for Data Analysis
- Addressing Contradictions : When conflicting data arise (e.g., conductivity values), conduct meta-analyses of published datasets, controlling for variables like measurement temperature and electrode configuration. Replicate experiments with strict protocol adherence and report uncertainty ranges .
- Reproducibility : Document synthesis conditions (precursor sources, furnace calibration) and instrument settings (e.g., spectrometer slit width) in detail. Share raw data and analysis scripts via repositories like Zenodo to enable independent verification .
Properties
IUPAC Name |
dicesium;oxygen(2-);titanium(4+) | |
---|---|---|
Source | PubChem | |
URL | https://pubchem.ncbi.nlm.nih.gov | |
Description | Data deposited in or computed by PubChem | |
InChI |
InChI=1S/2Cs.3O.Ti/q2*+1;3*-2;+4 | |
Source | PubChem | |
URL | https://pubchem.ncbi.nlm.nih.gov | |
Description | Data deposited in or computed by PubChem | |
InChI Key |
UKGZHELIUYCPTO-UHFFFAOYSA-N | |
Source | PubChem | |
URL | https://pubchem.ncbi.nlm.nih.gov | |
Description | Data deposited in or computed by PubChem | |
Canonical SMILES |
[O-2].[O-2].[O-2].[Ti+4].[Cs+].[Cs+] | |
Source | PubChem | |
URL | https://pubchem.ncbi.nlm.nih.gov | |
Description | Data deposited in or computed by PubChem | |
Molecular Formula |
Cs2O3Ti | |
Source | PubChem | |
URL | https://pubchem.ncbi.nlm.nih.gov | |
Description | Data deposited in or computed by PubChem | |
DSSTOX Substance ID |
DTXSID10923872 | |
Record name | Cesium titanium oxide (Cs2TiO3) | |
Source | EPA DSSTox | |
URL | https://comptox.epa.gov/dashboard/DTXSID10923872 | |
Description | DSSTox provides a high quality public chemistry resource for supporting improved predictive toxicology. | |
Molecular Weight |
361.676 g/mol | |
Source | PubChem | |
URL | https://pubchem.ncbi.nlm.nih.gov | |
Description | Data deposited in or computed by PubChem | |
CAS No. |
12158-57-5 | |
Record name | Cesium titanium oxide (Cs2TiO3) | |
Source | ChemIDplus | |
URL | https://pubchem.ncbi.nlm.nih.gov/substance/?source=chemidplus&sourceid=0012158575 | |
Description | ChemIDplus is a free, web search system that provides access to the structure and nomenclature authority files used for the identification of chemical substances cited in National Library of Medicine (NLM) databases, including the TOXNET system. | |
Record name | Cesium titanium oxide (Cs2TiO3) | |
Source | EPA DSSTox | |
URL | https://comptox.epa.gov/dashboard/DTXSID10923872 | |
Description | DSSTox provides a high quality public chemistry resource for supporting improved predictive toxicology. | |
Disclaimer and Information on In-Vitro Research Products
Please be aware that all articles and product information presented on BenchChem are intended solely for informational purposes. The products available for purchase on BenchChem are specifically designed for in-vitro studies, which are conducted outside of living organisms. In-vitro studies, derived from the Latin term "in glass," involve experiments performed in controlled laboratory settings using cells or tissues. It is important to note that these products are not categorized as medicines or drugs, and they have not received approval from the FDA for the prevention, treatment, or cure of any medical condition, ailment, or disease. We must emphasize that any form of bodily introduction of these products into humans or animals is strictly prohibited by law. It is essential to adhere to these guidelines to ensure compliance with legal and ethical standards in research and experimentation.