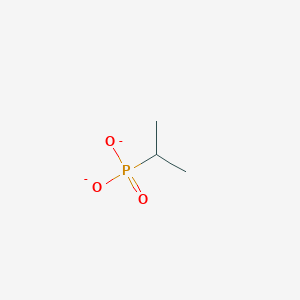
Dimethylmethanephosphonate
- Click on QUICK INQUIRY to receive a quote from our team of experts.
- With the quality product at a COMPETITIVE price, you can focus more on your research.
Overview
Description
Dimethylmethanephosphonate (DMMP), also known as dimethyl methylphosphonate (CAS 756-79-6), is an organophosphorus compound with the molecular formula C₃H₉O₃P and a molar mass of 124.08 g/mol . Structurally, it consists of a methyl group bonded to a phosphorus atom, which is further linked to two methoxy groups. DMMP is widely recognized as a flame retardant due to its ability to inhibit combustion by forming a protective char layer . Key properties include a density of 1.145 g/mL at 25°C and stability under standard conditions . Its synonyms include Methanephosphonic acid dimethyl ester and O,O-Dimethyl methylphosphonate .
Scientific Research Applications
Chemical Synthesis
Dimethylmethanephosphonate is widely used as a reagent in organic synthesis. It serves as an important intermediate for the production of phosphonates and phosphonic acid derivatives. These compounds are crucial in developing agrochemicals, pharmaceuticals, and flame retardants.
Case Study: Synthesis of Phosphonate Derivatives
A study demonstrated the use of this compound in synthesizing phosphonate derivatives through nucleophilic substitution reactions. The reaction conditions were optimized to enhance yield and selectivity, showcasing DMMP's role in producing high-value chemical intermediates .
Flame Retardants
One of the significant applications of this compound is in the formulation of flame retardants. Its phosphorus content enhances the flame resistance of various materials, including plastics and textiles.
Case Study: Flame Retardant Efficacy
Research conducted on the effects of this compound on methane flames revealed that it effectively reduces flame propagation rates. The study utilized a flat flame burner setup to assess combustion characteristics, confirming that DMMP enhances the thermal stability of materials while reducing smoke generation .
Environmental Applications
This compound has been studied for its potential environmental applications, particularly in the degradation of harmful substances. Its catalytic properties make it suitable for use in environmental remediation processes.
Case Study: Catalytic Decomposition
A study investigated the catalytic oxidative decomposition of this compound using various catalysts. The results indicated that DMMP could be effectively decomposed into less harmful substances under specific conditions, highlighting its potential role in detoxifying environments contaminated with nerve agents .
Biological Applications
Recent research has explored the biological implications of this compound, particularly its interactions with microbial communities. It is known to influence methane production in aquatic environments.
Case Study: Microbial Methane Production
A study examined how microbial communities utilize this compound to produce methane in oxic waters. The findings showed that DMMP could serve as a substrate for methane-producing microorganisms, contributing to greenhouse gas emissions under certain conditions .
Data Tables
Application Area | Description | Key Findings |
---|---|---|
Chemical Synthesis | Intermediate for phosphonates | High yields in optimized reactions |
Flame Retardants | Enhances thermal stability | Reduces flame propagation rates |
Environmental Remediation | Catalytic decomposition | Effective detoxification of harmful substances |
Biological Interactions | Methane production by microbes | Influences methane emissions in aquatic systems |
Q & A
Q. What safety protocols are essential when working with dimethylmethanephosphonate in laboratory settings?
Methodological Answer:
- Ventilation and PPE: Use fume hoods or local exhaust ventilation to avoid inhaling dust or vapors. Wear nitrile gloves, lab coats, and safety goggles to prevent skin/eye contact .
- Spill Management: Collect spills using non-sparking tools, avoid dust generation, and dispose of contaminated material in sealed containers. Environmental precautions include preventing drainage contamination .
- Decomposition Risks: During combustion or thermal degradation, toxic gases (e.g., phosphorus oxides, carbon monoxide) may form. Use water spray, CO₂, or dry chemical extinguishers .
Q. Which spectroscopic methods are commonly employed to confirm the structure of this compound derivatives?
Methodological Answer:
- Nuclear Magnetic Resonance (NMR):
- ¹H-NMR: Peaks at δ 1.30 (triplet, CH₃–CH₂–O) and δ 2.77 (singlet, N–CH₃) confirm alkyl and dimethylhydrazone groups .
- ¹³C-NMR: Signals at δ 16.4 (CH₃–CH₂–O) and δ 126.1 (C-1) validate phosphonate backbone and substituents .
- Mass Spectrometry (EI-MS): Key fragments at m/z 152 (base peak) and 125 (C–P bond cleavage) provide molecular ion patterns for structural verification .
Technique | Key Spectral Data | Functional Group Confirmation |
---|---|---|
¹H-NMR | δ 2.77 (s, 6H, N–CH₃) | Dimethylhydrazone protection |
¹³C-NMR | δ 126.1 (C-1) | Aldehyde-derived carbon |
EI-MS | m/z 222 (M⁺), 125 (100% intensity) | Molecular ion and fragmentation pathways |
Q. How can reaction conditions be optimized for synthesizing DMMP derivatives to improve yield and purity?
Methodological Answer:
- Catalyst Selection: Use p-toluenesulfonic acid (0.1–0.3 eq.) for efficient deprotection of acetal groups in acetone/water mixtures. Neutralize with NaHCO₃ post-reaction to avoid side reactions .
- Purification Techniques: Employ Kugelrohr distillation (62°C, 0.05 Torr) to isolate volatile derivatives. For non-volatile products, use column chromatography with phosphate buffer-methanol-acetonitrile (31:11:8) mobile phases .
- Reaction Monitoring: Track progress via GC or TLC to terminate reactions at optimal conversion (e.g., 48 hours for dimethylhydrazone formation) .
Q. What methodological approaches address discrepancies in reactivity data for DMMP under varying experimental conditions?
Methodological Answer:
- Controlled Parameter Testing: Use full factorial design to isolate variables (e.g., temperature, catalyst loading, solvent ratios). For example, LiN(iPr)₂ in THF at 25°C vs. elevated temperatures can reveal kinetic vs. thermodynamic control .
- Cross-Validation: Combine NMR, MS, and chromatographic data to resolve ambiguities. For instance, conflicting MS peaks may require high-resolution MS or isotopic labeling .
- Replication Studies: Repeat experiments under inert atmospheres (argon) to exclude moisture/oxygen interference, a common source of variability in phosphonate chemistry .
Q. How should researchers design experiments to analyze DMMP’s stability in aqueous environments?
Methodological Answer:
- pH-Dependent Studies: Prepare buffered solutions (pH 3–9) using monobasic potassium phosphate (6.8 g/L) adjusted with phosphoric acid. Monitor hydrolysis via ³¹P-NMR to track phosphonate ester cleavage .
- Accelerated Stability Testing: Use elevated temperatures (40–60°C) and quantify degradation products (e.g., methylphosphonic acid) via ion chromatography .
- Environmental Simulation: Model natural water systems by adding NaCl (25 g/L) to assess salinity effects on hydrolysis rates .
Q. What advanced techniques characterize the mechanistic pathways of DMMP in organocatalytic reactions?
Methodological Answer:
- Kinetic Isotope Effects (KIE): Compare reaction rates using deuterated vs. protiated substrates to identify rate-determining steps (e.g., hydride transfer vs. nucleophilic attack) .
- Computational Modeling: Apply DFT calculations (e.g., B3LYP/6-31G*) to map transition states in LiN(iPr)₂-mediated reactions, correlating with experimental NMR shifts .
- In Situ Spectroscopy: Use FT-IR to detect intermediate species (e.g., diazo compounds) during Bestmann-Ohira reagent reactions .
Comparison with Similar Compounds
Comparison with Similar Compounds
The following analysis compares DMMP with structurally and functionally related organophosphorus compounds, emphasizing molecular features, applications, and reactivity.
Diethyl Methanephosphonate (DEMP)
- CAS : 683-08-9
- Formula : C₅H₁₃O₃P
- Molecular Weight : 152.13 g/mol
- Key Differences :
- DEMP replaces DMMP’s methoxy groups with ethoxy substituents, increasing molecular weight and likely hydrophobicity.
- Applications: DEMP is less commonly used as a flame retardant but serves as a precursor in synthesizing pesticides or nerve agent analogs due to its higher alkyl chain flexibility .
- Reactivity: The ethyl groups may slow hydrolysis compared to DMMP’s methyl groups, altering its environmental persistence .
Dimethyl Phosphite
- CAS : 868-85-9
- Formula : C₂H₇O₃P
- Key Differences :
- Structural isomer of DMMP but classified as a phosphite (P–H bond) rather than a phosphonate (P–C bond).
- Reactivity: Phosphites are more reactive in esterification and transesterification reactions, making them valuable in polymer synthesis .
- Applications: Primarily used as a reducing agent or intermediate in agrochemical production, unlike DMMP’s flame-retardant role .
Diphenyl Methylphosphonate
- CAS : 7526-26-3
- Formula : C₁₃H₁₃O₃P
- Key Differences :
Diethyl (Methoxycarbonylmethyl)phosphonate
- CAS : 1596-84-5
- Formula : C₇H₁₅O₅P
- Key Differences :
Data Table: Structural and Functional Comparison
Compound | CAS | Formula | Molecular Weight (g/mol) | Key Applications | Reactivity Features |
---|---|---|---|---|---|
DMMP | 756-79-6 | C₃H₉O₃P | 124.08 | Flame retardant | Hydrolytically stable; char-forming |
DEMP | 683-08-9 | C₅H₁₃O₃P | 152.13 | Pesticide precursor | Slower hydrolysis; flexible alkyl chain |
Dimethyl Phosphite | 868-85-9 | C₂H₇O₃P | 110.05 | Reducing agent | High reactivity in esterification |
Diphenyl Methylphosphonate | 7526-26-3 | C₁₃H₁₃O₃P | 248.21 | High-temperature plastics | Enhanced thermal stability |
Flame Retardancy
DMMP’s efficacy as a flame retardant stems from its ability to decompose endothermically, releasing phosphoric acid derivatives that catalyze char formation . In contrast, DEMP’s larger alkyl groups reduce char-forming efficiency, limiting its utility in this domain .
Properties
Molecular Formula |
C3H7O3P-2 |
---|---|
Molecular Weight |
122.06 g/mol |
IUPAC Name |
dioxido-oxo-propan-2-yl-λ5-phosphane |
InChI |
InChI=1S/C3H9O3P/c1-3(2)7(4,5)6/h3H,1-2H3,(H2,4,5,6)/p-2 |
InChI Key |
ATLPLEZDTSBZQG-UHFFFAOYSA-L |
Canonical SMILES |
CC(C)P(=O)([O-])[O-] |
Origin of Product |
United States |
Disclaimer and Information on In-Vitro Research Products
Please be aware that all articles and product information presented on BenchChem are intended solely for informational purposes. The products available for purchase on BenchChem are specifically designed for in-vitro studies, which are conducted outside of living organisms. In-vitro studies, derived from the Latin term "in glass," involve experiments performed in controlled laboratory settings using cells or tissues. It is important to note that these products are not categorized as medicines or drugs, and they have not received approval from the FDA for the prevention, treatment, or cure of any medical condition, ailment, or disease. We must emphasize that any form of bodily introduction of these products into humans or animals is strictly prohibited by law. It is essential to adhere to these guidelines to ensure compliance with legal and ethical standards in research and experimentation.