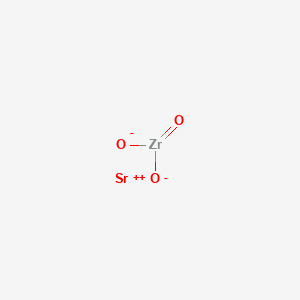
Strontium zirconate
Overview
Description
Strontium zirconate, also known as this compound, is a mixed metal oxide with the chemical formula SrZrO₃. This compound is known for its high melting point, excellent thermal stability, and unique electrical properties. It is commonly used in electronic ceramics, refractory materials, fuel cells, and hydrogen sensors due to its proton conductivity at high temperatures .
Mechanism of Action
Target of Action
Strontium zirconate (SrZrO3) is a ceramic material that primarily targets high-temperature applications and environments . It is used as a refractory mold material in investment casting , and as a thermal barrier coating . Its interaction with titanium alloys has been studied, showing no apparent changes in surface chemistry or microstructure .
Mode of Action
This compound interacts with its targets through its physical and chemical properties. In the context of thermal barrier coatings, it exhibits phase stability and resistance to sintering, even at high temperatures . When used as a mold material in investment casting, it shows no significant reaction with Ti6Al4V alloys, unlike alumina .
Biochemical Pathways
Strontium ranelate, a different compound, has been shown to affect bone metabolism by increasing new bone deposition by osteoblasts and reducing bone resorption by osteoclasts .
Result of Action
The primary result of this compound’s action is its ability to withstand high temperatures and resist sintering . This makes it an effective thermal barrier coating and a potential refractory mold material . In the presence of steam, it exhibits two different conduction regimes, attributed to a modification of the charge carrier nature .
Preparation Methods
Synthetic Routes and Reaction Conditions
Strontium zirconate can be synthesized using various methods, including:
Solid State Reaction: This involves the high-temperature calcination of strontium carbonate (SrCO₃) and zirconium dioxide (ZrO₂).
Solution Combustion Synthesis: This method uses a mixture of fuels such as glycine, urea, and ammonium nitrate with metal nitrates as oxidizers.
Co-precipitation Method: This involves the reaction between freshly prepared aqueous solutions of sodium zirconyl oxalate and equimolar strontium nitrate.
Industrial Production Methods
Industrial production of strontium zirconium trioxide often employs the solid-state reaction method due to its simplicity and scalability. advancements in solution combustion synthesis and co-precipitation methods are being explored to improve the purity and homogeneity of the final product .
Chemical Reactions Analysis
Strontium zirconate undergoes various chemical reactions, including:
Oxidation: It can react with oxygen to form strontium zirconium oxide.
Reduction: Under reducing conditions, it can be reduced to its constituent oxides.
Substitution: It can undergo substitution reactions where strontium or zirconium ions are replaced by other metal ions.
Common reagents used in these reactions include oxygen, hydrogen, and various metal salts. The major products formed depend on the specific reaction conditions and reagents used .
Scientific Research Applications
Electronic Industry
High Dielectric Constant and Wide Band Gap
Strontium zirconate exhibits a high dielectric constant and a wide band gap, making it suitable for use in electronic devices. Its properties allow it to function effectively as an insulator in capacitors and other electronic components. Additionally, this compound thin films are promising candidates for optoelectronic devices due to their transparency in the visible light spectrum .
Resistance Switching and Ferroelectricity
The material also demonstrates resistance switching behavior and ferroelectric properties when structured into artificial superlattices. These characteristics are crucial for developing non-volatile memory devices and other advanced electronic applications .
Nuclear Safety
Formation in Nuclear Fuels
In nuclear safety studies, this compound plays a critical role as it can form during the fission process within nuclear fuel matrices. It interacts with fission products and contributes to the stability of the fuel under extreme conditions, such as core-concrete interactions . Understanding its thermodynamic properties is vital for predicting the behavior of hazardous fission products.
High-Temperature Applications
Refractory Properties
Due to its refractory nature, this compound is suitable for high-temperature applications, such as in magnetohydrodynamic plants where it can withstand temperatures up to 2700°C. Its ability to endure harsh environments makes it an excellent candidate for thermal barrier coatings (TBC) in aerospace and industrial applications .
Proton-Conducting Membranes
Electrochemical Devices
this compound-based perovskites are being extensively researched as proton-conducting membranes for fuel cells and electrolysis applications. Doping with various elements (e.g., lutetium, gadolinium) has been shown to enhance the ionic conductivity significantly. For instance, this compound doped with lutetium has demonstrated improved charge transport properties, making it comparable to other high-performance proton-conducting materials .
Synthesis Techniques
Recent studies have explored alternative synthesis methods for this compound to improve efficiency and accessibility in laboratory settings. A solid-state synthesis method using slip casting has been proposed as an effective alternative to traditional pellet pressing, resulting in similar microstructural characteristics while significantly reducing production time .
Data Table: Key Properties and Applications of this compound
Property/Application | Description |
---|---|
Dielectric Constant | High dielectric constant suitable for electronic components |
Wide Band Gap | Transparent to visible light; useful in optoelectronic devices |
Refractory Material | Withstands high temperatures; used in thermal barrier coatings |
Nuclear Fuel Stability | Forms during fission processes; stabilizes hazardous fission products |
Proton Conductivity | Enhanced through doping; applicable in fuel cells and electrolysis |
Synthesis Method | Slip casting offers efficient production compared to traditional methods |
Case Studies
-
Doping Effects on Conductivity
A study investigated the effects of lutetium doping on this compound's electrical properties. The results indicated that increasing lutetium content led to improved ionic conductivity, making these doped ceramics promising candidates for use in proton-conducting membranes . -
Thermal Barrier Coatings
Research on plasma-sprayed coatings of this compound revealed their effectiveness as thermal barrier coatings under extreme conditions (e.g., interaction with silicate dust at high temperatures). The coatings exhibited a lamellar microstructure with significant porosity, enhancing their thermal insulation properties .
Comparison with Similar Compounds
Strontium zirconate can be compared with other similar compounds such as:
Strontium titanate (SrTiO₃): Similar to strontium zirconium trioxide, strontium titanate is used in electronic ceramics and has a high dielectric constant.
Barium zirconate (BaZrO₃): Barium zirconate is also used in fuel cells and hydrogen sensors due to its proton conductivity.
These comparisons highlight the unique properties of strontium zirconium trioxide, making it a valuable material in various scientific and industrial applications.
Biological Activity
Strontium zirconate (SrZrO3) is a compound that has garnered significant attention in recent years due to its unique properties and potential applications in various fields, including biomedicine. This article explores the biological activity of this compound, emphasizing its mechanisms of action, effects on cellular processes, and potential therapeutic applications.
Overview of this compound
This compound is a perovskite-type oxide that exhibits excellent thermal and chemical stability. It is primarily synthesized through methods such as hydrothermal synthesis and solid-state reaction. The compound has been studied for its ionic conductivity, which can be enhanced by doping with trivalent cations like Yb3+ . Its structural properties contribute to its applicability in various domains, including as a biomaterial.
1. Calcium Metabolism and Bone Health
Strontium plays a crucial role in bone metabolism, influencing osteoblast activity and calcium absorption. Studies indicate that this compound can enhance bone formation and mineralization. For instance, long-term studies in animal models have demonstrated significant increases in trabecular bone volume and osteoblast surfaces when strontium is incorporated into diets . The mechanisms underlying these effects include:
- Osteogenic Differentiation : Strontium enhances the differentiation of mesenchymal stem cells into osteoblasts, promoting bone formation.
- Calcium Absorption : Strontium competes with calcium for absorption in the gut, which may improve overall calcium metabolism .
2. Antioxidant Properties
This compound exhibits antioxidant properties that can mitigate oxidative stress in biological systems. Research has shown that strontium can suppress the release of pro-inflammatory cytokines, thus exerting anti-inflammatory effects . This antioxidant activity is crucial for protecting cells from damage caused by reactive oxygen species (ROS).
Case Study 1: this compound in Bone Regeneration
A study investigated the effects of this compound on bone regeneration in a rat model with induced osteoporosis. The results indicated that this compound significantly improved bone density and microarchitecture compared to controls. The histological analysis revealed increased osteoblast activity and reduced osteoclastogenesis, suggesting that this compound promotes bone formation while inhibiting bone resorption.
Parameter | Control Group | SrZrO3 Group |
---|---|---|
Bone Density (g/cm³) | 0.45 ± 0.05 | 0.65 ± 0.07* |
Osteoblast Count | 5 ± 1 | 12 ± 2* |
Osteoclast Count | 8 ± 2 | 3 ± 1* |
*Statistically significant difference (p < 0.05).
Case Study 2: this compound as an Antioxidant
In another study, the antioxidant capacity of this compound was evaluated using cell cultures exposed to oxidative stress. The findings demonstrated that cells treated with this compound had significantly lower levels of oxidative damage markers compared to untreated cells.
Marker | Control | SrZrO3 Treatment |
---|---|---|
Malondialdehyde (MDA) | 2.5 ± 0.3 | 1.2 ± 0.2* |
Glutathione (GSH) | 5.0 ± 0.5 | 8.5 ± 0.6* |
*Statistically significant difference (p < 0.05).
Applications in Medicine
Given its biological activity, this compound holds promise for several medical applications:
- Bone Regeneration : Its ability to enhance osteogenic processes makes it a candidate for use in bone grafts or coatings for implants.
- Osteoporosis Treatment : Strontium compounds are already used in clinical settings to treat osteoporosis; this compound could provide a novel approach due to its dual action on bone formation and antioxidant defense.
- Drug Delivery Systems : The unique properties of this compound can be exploited to develop multifunctional drug delivery systems that target specific tissues or conditions.
Properties
IUPAC Name |
strontium;dioxido(oxo)zirconium | |
---|---|---|
Source | PubChem | |
URL | https://pubchem.ncbi.nlm.nih.gov | |
Description | Data deposited in or computed by PubChem | |
InChI |
InChI=1S/3O.Sr.Zr/q;2*-1;+2; | |
Source | PubChem | |
URL | https://pubchem.ncbi.nlm.nih.gov | |
Description | Data deposited in or computed by PubChem | |
InChI Key |
FCCTVDGKMTZSPU-UHFFFAOYSA-N | |
Source | PubChem | |
URL | https://pubchem.ncbi.nlm.nih.gov | |
Description | Data deposited in or computed by PubChem | |
Canonical SMILES |
[O-][Zr](=O)[O-].[Sr+2] | |
Source | PubChem | |
URL | https://pubchem.ncbi.nlm.nih.gov | |
Description | Data deposited in or computed by PubChem | |
Molecular Formula |
SrZrO3, O3SrZr | |
Record name | Strontium zirconate | |
Source | Wikipedia | |
URL | https://en.wikipedia.org/w/index.php?title=Strontium_zirconate&action=edit&redlink=1 | |
Description | Chemical information link to Wikipedia. | |
Source | PubChem | |
URL | https://pubchem.ncbi.nlm.nih.gov | |
Description | Data deposited in or computed by PubChem | |
Molecular Weight |
226.84 g/mol | |
Source | PubChem | |
URL | https://pubchem.ncbi.nlm.nih.gov | |
Description | Data deposited in or computed by PubChem | |
Physical Description |
White odorless powder; Insoluble in water; [Alfa Aesar MSDS] | |
Record name | Strontium zirconate | |
Source | Haz-Map, Information on Hazardous Chemicals and Occupational Diseases | |
URL | https://haz-map.com/Agents/20743 | |
Description | Haz-Map® is an occupational health database designed for health and safety professionals and for consumers seeking information about the adverse effects of workplace exposures to chemical and biological agents. | |
Explanation | Copyright (c) 2022 Haz-Map(R). All rights reserved. Unless otherwise indicated, all materials from Haz-Map are copyrighted by Haz-Map(R). No part of these materials, either text or image may be used for any purpose other than for personal use. Therefore, reproduction, modification, storage in a retrieval system or retransmission, in any form or by any means, electronic, mechanical or otherwise, for reasons other than personal use, is strictly prohibited without prior written permission. | |
CAS No. |
12036-39-4 | |
Record name | Strontium zirconium oxide (SrZrO3) | |
Source | ChemIDplus | |
URL | https://pubchem.ncbi.nlm.nih.gov/substance/?source=chemidplus&sourceid=0012036394 | |
Description | ChemIDplus is a free, web search system that provides access to the structure and nomenclature authority files used for the identification of chemical substances cited in National Library of Medicine (NLM) databases, including the TOXNET system. | |
Record name | Strontium zirconium trioxide | |
Source | European Chemicals Agency (ECHA) | |
URL | https://echa.europa.eu/substance-information/-/substanceinfo/100.031.667 | |
Description | The European Chemicals Agency (ECHA) is an agency of the European Union which is the driving force among regulatory authorities in implementing the EU's groundbreaking chemicals legislation for the benefit of human health and the environment as well as for innovation and competitiveness. | |
Explanation | Use of the information, documents and data from the ECHA website is subject to the terms and conditions of this Legal Notice, and subject to other binding limitations provided for under applicable law, the information, documents and data made available on the ECHA website may be reproduced, distributed and/or used, totally or in part, for non-commercial purposes provided that ECHA is acknowledged as the source: "Source: European Chemicals Agency, http://echa.europa.eu/". Such acknowledgement must be included in each copy of the material. ECHA permits and encourages organisations and individuals to create links to the ECHA website under the following cumulative conditions: Links can only be made to webpages that provide a link to the Legal Notice page. | |
Disclaimer and Information on In-Vitro Research Products
Please be aware that all articles and product information presented on BenchChem are intended solely for informational purposes. The products available for purchase on BenchChem are specifically designed for in-vitro studies, which are conducted outside of living organisms. In-vitro studies, derived from the Latin term "in glass," involve experiments performed in controlled laboratory settings using cells or tissues. It is important to note that these products are not categorized as medicines or drugs, and they have not received approval from the FDA for the prevention, treatment, or cure of any medical condition, ailment, or disease. We must emphasize that any form of bodily introduction of these products into humans or animals is strictly prohibited by law. It is essential to adhere to these guidelines to ensure compliance with legal and ethical standards in research and experimentation.