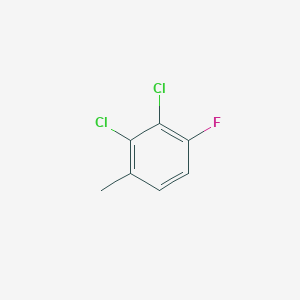
2,3-Dichloro-4-fluorotoluene
Overview
Description
2,3-Dichloro-4-fluorotoluene is an aromatic compound with the molecular formula C7H5Cl2F It is a derivative of benzene, where the benzene ring is substituted with two chlorine atoms, one fluorine atom, and one methyl group
Preparation Methods
Synthetic Routes and Reaction Conditions: The synthesis of 2,3-Dichloro-4-fluorotoluene typically involves electrophilic aromatic substitution reactions. One common method is the chlorination and fluorination of 4-methylbenzene (toluene). The process involves the following steps:
Chlorination: Toluene is first chlorinated using chlorine gas in the presence of a catalyst such as ferric chloride (FeCl3) to introduce chlorine atoms at the 2 and 3 positions.
Fluorination: The chlorinated intermediate is then subjected to fluorination using a fluorinating agent like hydrogen fluoride (HF) or a fluorine gas under controlled conditions to introduce the fluorine atom at the 1 position.
Industrial Production Methods: Industrial production of this compound follows similar synthetic routes but on a larger scale. The reaction conditions are optimized to ensure high yield and purity of the final product. The use of continuous flow reactors and advanced separation techniques helps in achieving efficient production.
Chemical Reactions Analysis
Types of Reactions: 2,3-Dichloro-4-fluorotoluene undergoes various chemical reactions, including:
Substitution Reactions: It can undergo nucleophilic substitution reactions where the chlorine or fluorine atoms are replaced by other nucleophiles.
Oxidation Reactions: The methyl group can be oxidized to form corresponding carboxylic acids or aldehydes.
Reduction Reactions: The compound can be reduced to form different derivatives depending on the reducing agents used.
Common Reagents and Conditions:
Nucleophilic Substitution: Reagents like sodium hydroxide (NaOH) or potassium hydroxide (KOH) in aqueous or alcoholic medium.
Oxidation: Oxidizing agents such as potassium permanganate (KMnO4) or chromium trioxide (CrO3) in acidic medium.
Reduction: Reducing agents like lithium aluminum hydride (LiAlH4) or hydrogen gas (H2) in the presence of a catalyst.
Major Products:
Substitution Products: Depending on the nucleophile, products like 2,3-dichloro-1-hydroxy-4-methylbenzene or 2,3-dichloro-1-amino-4-methylbenzene can be formed.
Oxidation Products: Products like 2,3-dichloro-1-fluoro-4-methylbenzoic acid or 2,3-dichloro-1-fluoro-4-methylbenzaldehyde.
Reduction Products: Products like 2,3-dichloro-1-fluoro-4-methylcyclohexane.
Scientific Research Applications
2,3-Dichloro-4-fluorotoluene has several applications in scientific research:
Chemistry: It is used as an intermediate in the synthesis of more complex organic compounds. Its unique substitution pattern makes it a valuable building block in organic synthesis.
Biology: The compound is studied for its potential biological activity. Derivatives of this compound are investigated for their antimicrobial and anticancer properties.
Medicine: Research is ongoing to explore its potential as a pharmaceutical intermediate. It may serve as a precursor for the synthesis of drugs with specific therapeutic effects.
Industry: It is used in the production of agrochemicals, dyes, and polymers. Its stability and reactivity make it suitable for various industrial applications.
Mechanism of Action
The mechanism of action of 2,3-Dichloro-4-fluorotoluene depends on its interaction with specific molecular targets. In biological systems, it may interact with enzymes or receptors, leading to inhibition or activation of specific pathways. The exact mechanism varies depending on the derivative and the target system. For example, in antimicrobial studies, it may disrupt cell membrane integrity or interfere with essential metabolic processes.
Comparison with Similar Compounds
- 2,4-Dichloro-1-fluoro-4-methylbenzene
- 2,3-Dichloro-1-fluoro-5-methylbenzene
- 2,3-Dichloro-1-fluoro-4-ethylbenzene
Comparison: 2,3-Dichloro-4-fluorotoluene is unique due to its specific substitution pattern, which influences its chemical reactivity and physical properties. Compared to similar compounds, it may exhibit different reactivity in substitution reactions or different biological activity. The presence of both chlorine and fluorine atoms in specific positions can lead to unique electronic effects, making it distinct from other derivatives.
Properties
Molecular Formula |
C7H5Cl2F |
---|---|
Molecular Weight |
179.02 g/mol |
IUPAC Name |
2,3-dichloro-1-fluoro-4-methylbenzene |
InChI |
InChI=1S/C7H5Cl2F/c1-4-2-3-5(10)7(9)6(4)8/h2-3H,1H3 |
InChI Key |
HIXXDQPJVORCDB-UHFFFAOYSA-N |
Canonical SMILES |
CC1=C(C(=C(C=C1)F)Cl)Cl |
Origin of Product |
United States |
Synthesis routes and methods
Procedure details
Disclaimer and Information on In-Vitro Research Products
Please be aware that all articles and product information presented on BenchChem are intended solely for informational purposes. The products available for purchase on BenchChem are specifically designed for in-vitro studies, which are conducted outside of living organisms. In-vitro studies, derived from the Latin term "in glass," involve experiments performed in controlled laboratory settings using cells or tissues. It is important to note that these products are not categorized as medicines or drugs, and they have not received approval from the FDA for the prevention, treatment, or cure of any medical condition, ailment, or disease. We must emphasize that any form of bodily introduction of these products into humans or animals is strictly prohibited by law. It is essential to adhere to these guidelines to ensure compliance with legal and ethical standards in research and experimentation.