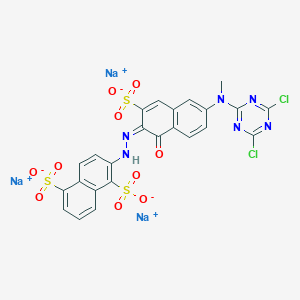
C.I. Reactive Orange 4
Overview
Description
C.I. Reactive Orange 4 (CAS 70616-90-9) is a sulfonated azo-reactive dye with the molecular formula C₂₄H₁₇Cl₂N₆NaO₁₀S₃ and a molar mass of 739.5 g/mol . Its chemical structure (Figure 1) consists of a 1,3,5-triazine ring substituted with two chlorine atoms and linked to a naphthalene sulfonate backbone via an azo (-N=N-) bond . This dye is water-soluble and widely used in textile industries for dyeing cotton, nylon, silk, and wool due to its bright orange hue and covalent bonding capability with cellulose fibers . Production involves diazotization of 2-amino-1,5-naphthalenedisulfonic acid, followed by coupling with N-methyl J acid and subsequent chlorination using cobalt hypochlorite .
Preparation Methods
Synthetic Routes and Reaction Conditions
The synthesis of C.I. Reactive Orange 4 involves the diazotization of 2-naphthylamine-1,5-disulfonic acid followed by coupling with 2,4,6-trichloro-1,3,5-triazine and 5-hydroxy-2-(N-methyl) naphthylamine-7-sulfonic acid . The reaction conditions typically include maintaining a low temperature to ensure the stability of the diazonium salt and using an alkaline medium for the coupling reaction.
Industrial Production Methods
In industrial settings, the production of C.I. Reactive Orange 4 is carried out in large-scale reactors where precise control of temperature, pH, and reactant concentrations is maintained. The process involves continuous monitoring and adjustment to optimize yield and purity. The final product is usually isolated by filtration, washed, and dried before being packaged for use.
Chemical Reactions Analysis
Reduction Reactions
C.I. Reactive Orange 4 undergoes reductive cleavage of its azo (-N=N- ) bonds, a critical pathway in environmental remediation:
-
Primary Agents : Sodium dithionite (Na₂S₂O₄) and zinc dust are commonly used .
-
Mechanism : The azo group is reduced to aromatic amines, forming intermediates such as 2-amino-1,5-naphthalenedisulphonic acid .
-
Electrochemical Reduction : Under galvanostatic conditions (stainless steel cathode, Na₂SO₄ electrolyte), the dye is decolorized with pseudo-first-order kinetics. A key intermediate identified via HPLC and XPS analysis matches the structure of 2-amino-1,5-naphthalenedisulphonic acid .
Key Reaction Pathway :
Oxidation Reactions
Oxidative degradation is critical for wastewater treatment:
-
Agents : Hydrogen peroxide (H₂O₂), potassium permanganate (KMnO₄), and electrochemical oxidation .
-
Electrochemical Oxidation : Using a Ti/SnO₂-Sb-Pt anode, both azo bonds and aromatic rings are cleaved, leading to complete mineralization .
-
Photocatalytic Oxidation : TiO₂ (Degussa P-25) under UV light degrades hydrolyzed and unhydrolysed forms of the dye. At pH 10, decolorization rates reach 90% within 20 minutes .
Kinetic Data for Photocatalytic Decolorization :
Solution Type | Catalyst (TiO₂) | pH | Rate Constant (min⁻¹) |
---|---|---|---|
Aqueous (38 mg/L) | Degussa P-25 | 5.6 | 0.0702 |
Aqueous (38 mg/L) | Degussa P-25 | 10 | 0.0909 |
Source: |
Substitution Reactions
The triazine ring in this compound facilitates nucleophilic substitution, critical for textile dye fixation:
-
Cellulose Binding : Hydroxyl groups on cellulose fibers react with the triazinyl chlorine atoms, forming covalent ether bonds under alkaline conditions .
-
Competitive Hydrolysis : In aqueous alkaline environments, hydroxide ions displace chlorine atoms, producing non-reactive hydrolyzed dye (15–40% in industrial effluents) .
Hydrolysis Reaction :
Photocatalytic Degradation Pathways
Heterogeneous photocatalysis using TiO₂ is highly effective:
-
Mechanism : UV/sunlight irradiation generates hydroxyl radicals (·OH) that oxidize the azo bond and aromatic rings.
-
Catalyst Efficiency : Degussa P-25 TiO₂ outperforms Riedel-de-Häen TiO₂ in simulated dyebath wastewater, achieving >90% decolorization .
-
pH Dependency : Rate constants increase by ~30% when pH rises from 5.6 to 10 .
Electrochemical Degradation
Galvanostatic treatment combines reduction and oxidation:
-
Anode (Oxidation) : Complete degradation of aromatic structures.
-
Cathode (Reduction) : Partial cleavage to amines.
-
Kinetics : Pseudo-first-order with rate constants dependent on current density .
Environmental and Biological Interactions
Scientific Research Applications
C.I. Reactive Orange 4 has several applications in scientific research:
Chemistry: Used as a model compound in studies of azo dye degradation and photocatalysis.
Biology: Employed in staining techniques to visualize cellular components.
Medicine: Investigated for its potential use in photodynamic therapy and as a diagnostic tool.
Industry: Widely used in textile dyeing, wastewater treatment studies, and as a tracer in environmental monitoring.
Mechanism of Action
The primary mechanism of action of C.I. Reactive Orange 4 involves its ability to form covalent bonds with hydroxyl groups on cellulose fibers. This reaction occurs through nucleophilic substitution on the triazine ring, resulting in a strong and durable attachment of the dye to the fiber. The molecular targets include the hydroxyl groups on cellulose, and the pathways involve the formation of a reactive intermediate that facilitates the bonding process .
Comparison with Similar Compounds
Comparison with Similar Compounds
The following table compares C.I. Reactive Orange 4 with structurally or functionally analogous reactive dyes:
Structural and Functional Differences:
Triazine Substitution :
- This compound and 5 both contain dichlorotriazine groups, enabling covalent bonding with cellulose. However, Orange 4 has two chlorine atoms on the triazine ring, whereas Orange 5 includes an additional sulfophenyl group .
- C.I. Reactive Orange 35 uses a tetrasulfonated benzenedisulfonic acid backbone, enhancing water solubility but increasing wastewater COD .
Degradation Pathways :
- Electrochemical oxidation of this compound generates hydroxyl radicals (·OH) that cleave the azo bond, leading to intermediates like sulfonated aromatic amines . In contrast, C.I. Reactive Orange 107 (structurally similar) undergoes faster mineralization (36% TOC removal) due to Gd³⁺/Nd³⁺-doped CeO₂ catalysts promoting superoxide radical (O₂⁻) formation .
Pigment vs. Reactive Dye: C.I. Pigment Orange 4 lacks sulfonate groups and is insoluble, making it suitable for non-textile applications like paints. Its azo bond is stabilized by methoxy and hydroxyl groups, improving lightfastness but limiting biodegradability .
Research Findings and Data
Electrochemical Degradation Efficiency:
Stability Under Environmental Conditions:
Biological Activity
C.I. Reactive Orange 4 (RO4) is a synthetic azo dye widely utilized in the textile industry. Its biological activity, particularly in relation to environmental impact and potential applications in bioremediation, has garnered significant attention. This article explores the biological activity of RO4, focusing on its degradation mechanisms, toxicity, and potential applications.
Overview of this compound
This compound is characterized by its azo (-N=N-) functional groups, which contribute to its vivid orange color and reactivity with cellulosic fibers. The compound's molecular weight is approximately 781.5 g/mol, and it is known for its excellent fastness properties in dyeing processes.
The primary mechanism of action for this compound involves the formation of covalent bonds with hydroxyl groups on cellulose fibers through nucleophilic substitution reactions on the triazine ring. This reaction results in a strong attachment of the dye to the fiber, enhancing its durability.
Toxicological Profile
Azo dyes, including RO4, are known for their potential toxic effects due to their ability to bioaccumulate in the environment. Studies have indicated that these compounds can exhibit carcinogenic and mutagenic properties, necessitating effective degradation strategies to mitigate their environmental impact .
Biodegradation Studies
Recent research has highlighted various biological agents capable of degrading this compound:
- Actinobacteria : Species such as Streptomyces albidoflavus have demonstrated significant capabilities in decolorizing and degrading azo dyes, including RO4. In one study, S. albidoflavus achieved a decolorization rate of over 60% within five days under optimized conditions (pH 6, temperature 35 °C) .
- Marine Actinobacteria : Another study isolated Nocardiopsis sp., which showed an impressive decolorization efficiency of 85.6% within 24 hours at optimal conditions (pH 8, temperature 35 °C) .
Electrochemical Degradation
Electrochemical methods have also been explored for the degradation of this compound. A study utilizing a silver-carbon composite electrode reported effective decolorization through electrochemical oxidation techniques, demonstrating an alternative approach for treating textile effluents containing RO4 .
Comparative Analysis of Degradation Techniques
Method | Organism/Agent | Decolorization Efficiency | Time Frame |
---|---|---|---|
Biodegradation | Streptomyces albidoflavus | Up to 60% | 5 days |
Biodegradation | Nocardiopsis sp. | 85.6% | 24 hours |
Electrochemical Oxidation | Silver-Carbon Composite | High | Varies |
Research Findings
- Biodegradation Mechanisms : The degradation pathways often involve enzymatic activity, with laccase enzymes playing a crucial role in breaking down the dye structure into less toxic metabolites .
- Environmental Impact : The biodegradation products from RO4 are typically less toxic than the parent compound, indicating that bioremediation strategies using microorganisms can effectively reduce environmental hazards associated with azo dyes .
- Application in Photodynamic Therapy : Preliminary studies suggest potential applications of RO4 in medical fields such as photodynamic therapy due to its ability to generate reactive oxygen species upon light activation.
Q & A
Basic Research Questions
Q. What spectroscopic and chromatographic methods are recommended for characterizing the structural integrity of C.I. Reactive Orange 4 before and after electrochemical degradation?
- Methodological Answer : Use Fourier-transform infrared spectroscopy (FTIR) to identify functional group changes (e.g., -N=N- bond cleavage) and gas chromatography-mass spectrometry (GC-MS) to detect intermediate compounds like sulfonated aromatics and phenols. For structural confirmation, nuclear magnetic resonance (NMR) can validate bond rearrangements .
Q. How should researchers design experiments to compare electro-oxidation (EO) and electrocatalytic oxidation (ECO) for this compound degradation?
- Methodological Answer :
- Variables : Fix pH at 9.4 and NaCl concentration at 0.08 M (to simulate common industrial effluent conditions).
- Catalysts : Use Sm³⁺/Gd³⁺/Nd³⁺-doped cerium oxide electrodes for ECO.
- Metrics : Measure total organic carbon (TOC) removal efficiency over 20 minutes (EO: ~24%, ECO: ~36%) and track reactive oxygen species (ROS) like O₂⁻ via radical scavenger tests .
Q. What are the key parameters for optimizing dye degradation efficiency in batch reactor systems?
- Methodological Answer : Prioritize pH (9.0–10.0 for ROS stability), electrolyte composition (Cl⁻ concentration for hypochlorite generation), and current density (10–30 mA/cm²). Validate via response surface methodology (RSM) to identify interactions between variables .
Advanced Research Questions
Q. How do intermediate degradation products of this compound influence reaction kinetics and toxicity profiles?
- Methodological Answer :
- Pathway Analysis : Use GC-MS to identify intermediates like N-(3-aminophenyl) acetamide and benzene-1,3-diamine. Quantify their persistence via high-performance liquid chromatography (HPLC).
- Toxicity : Assess acute toxicity using Daphnia magna bioassays. Note that phenol derivatives may exhibit higher toxicity than parent dyes, requiring extended oxidation for full mineralization .
Q. How can researchers resolve contradictions in reported TOC removal efficiencies for this compound across electrochemical studies?
- Methodological Answer :
- Systematic Comparison : Replicate studies under identical conditions (pH, catalyst loading, current density).
- Data Normalization : Express results as TOC removal per unit energy consumed (kWh/g TOC) to account for operational variability.
- Contradiction Source : Discrepancies often arise from differences in ROS generation mechanisms (e.g., direct electron transfer vs. indirect ·OH-mediated oxidation) .
Q. What mechanistic insights can be gained from analyzing the role of rare-earth metal dopants (Gd³⁺, Nd³⁺) in electrocatalytic oxidation?
- Methodological Answer :
- Surface Analysis : Use X-ray photoelectron spectroscopy (XPS) to confirm dopant integration into the CeO₂ lattice.
- Electrochemical Profiling : Conduct cyclic voltammetry (CV) to correlate dopant concentration with charge-transfer efficiency. Gd³⁺ enhances O₂⁻ generation via oxygen vacancy formation, while Nd³⁺ improves conductivity .
Q. How can researchers validate the repeatability of this compound degradation studies across laboratories?
- Methodological Answer :
- Protocol Standardization : Share detailed electrode fabrication steps (e.g., sol-gel synthesis parameters for doped catalysts) and ROS quantification methods.
- Reference Materials : Use a certified dye solution (e.g., 100 mg/L in 0.08 M NaCl) to calibrate reactors. Report uncertainties in TOC measurements (±5% via triplicate runs) .
Q. Data Analysis and Interpretation
Q. What statistical approaches are recommended for analyzing non-linear relationships in dye degradation kinetics?
- Methodological Answer : Apply pseudo-first-order or Langmuir-Hinshelwood models to fit kinetic data. Use ANOVA to assess significance of variables (e.g., pH vs. catalyst type). For non-linear systems, machine learning algorithms (e.g., random forest) can predict optimal conditions .
Q. How should researchers address discrepancies between FTIR and GC-MS data when identifying degradation intermediates?
- Methodological Answer : Cross-validate results by spiking samples with reference standards (e.g., sulfonated aromatics). If FTIR detects residual -SO₃ groups but GC-MS does not, consider matrix effects or ionization inefficiencies in GC-MS .
Q. Experimental Design and Validation
Q. What controls are essential for ensuring the reliability of electrochemical degradation studies?
- Methodological Answer :
- Blank Experiments : Run trials without current or catalyst to rule out photolytic/adsorption effects.
- Negative Controls : Use inert electrolytes (e.g., Na₂SO₄ instead of NaCl) to isolate ROS contributions.
- Replicates : Perform triplicate runs with fresh dye solutions to assess reproducibility .
Properties
IUPAC Name |
trisodium;2-[[6-[(4,6-dichloro-1,3,5-triazin-2-yl)-methylamino]-1-hydroxy-3-sulfonatonaphthalen-2-yl]diazenyl]naphthalene-1,5-disulfonate | |
---|---|---|
Source | PubChem | |
URL | https://pubchem.ncbi.nlm.nih.gov | |
Description | Data deposited in or computed by PubChem | |
InChI |
InChI=1S/C24H16Cl2N6O10S3.3Na/c1-32(24-28-22(25)27-23(26)29-24)12-5-6-13-11(9-12)10-18(44(37,38)39)19(20(13)33)31-30-16-8-7-14-15(21(16)45(40,41)42)3-2-4-17(14)43(34,35)36;;;/h2-10,33H,1H3,(H,34,35,36)(H,37,38,39)(H,40,41,42);;;/q;3*+1/p-3 | |
Source | PubChem | |
URL | https://pubchem.ncbi.nlm.nih.gov | |
Description | Data deposited in or computed by PubChem | |
InChI Key |
ARXWHYHTMGGNST-UHFFFAOYSA-K | |
Source | PubChem | |
URL | https://pubchem.ncbi.nlm.nih.gov | |
Description | Data deposited in or computed by PubChem | |
Canonical SMILES |
CN(C1=CC2=CC(=C(C(=C2C=C1)O)N=NC3=C(C4=C(C=C3)C(=CC=C4)S(=O)(=O)[O-])S(=O)(=O)[O-])S(=O)(=O)[O-])C5=NC(=NC(=N5)Cl)Cl.[Na+].[Na+].[Na+] | |
Source | PubChem | |
URL | https://pubchem.ncbi.nlm.nih.gov | |
Description | Data deposited in or computed by PubChem | |
Molecular Formula |
C24H13Cl2N6Na3O10S3 | |
Source | PubChem | |
URL | https://pubchem.ncbi.nlm.nih.gov | |
Description | Data deposited in or computed by PubChem | |
Related CAS |
73816-75-8 (Parent) | |
Record name | C.I. Reactive Orange 4 | |
Source | ChemIDplus | |
URL | https://pubchem.ncbi.nlm.nih.gov/substance/?source=chemidplus&sourceid=0070616909 | |
Description | ChemIDplus is a free, web search system that provides access to the structure and nomenclature authority files used for the identification of chemical substances cited in National Library of Medicine (NLM) databases, including the TOXNET system. | |
DSSTOX Substance ID |
DTXSID4072079 | |
Record name | C.I. Reactive Orange 4 | |
Source | EPA DSSTox | |
URL | https://comptox.epa.gov/dashboard/DTXSID4072079 | |
Description | DSSTox provides a high quality public chemistry resource for supporting improved predictive toxicology. | |
Molecular Weight |
781.5 g/mol | |
Source | PubChem | |
URL | https://pubchem.ncbi.nlm.nih.gov | |
Description | Data deposited in or computed by PubChem | |
CAS No. |
70616-90-9, 12225-82-0 | |
Record name | C.I. Reactive Orange 4 | |
Source | ChemIDplus | |
URL | https://pubchem.ncbi.nlm.nih.gov/substance/?source=chemidplus&sourceid=0070616909 | |
Description | ChemIDplus is a free, web search system that provides access to the structure and nomenclature authority files used for the identification of chemical substances cited in National Library of Medicine (NLM) databases, including the TOXNET system. | |
Record name | 1,5-Naphthalenedisulfonic acid, 2-[2-[6-[(4,6-dichloro-1,3,5-triazin-2-yl)methylamino]-1-hydroxy-3-sulfo-2-naphthalenyl]diazenyl]-, sodium salt (1:3) | |
Source | EPA Chemicals under the TSCA | |
URL | https://www.epa.gov/chemicals-under-tsca | |
Description | EPA Chemicals under the Toxic Substances Control Act (TSCA) collection contains information on chemicals and their regulations under TSCA, including non-confidential content from the TSCA Chemical Substance Inventory and Chemical Data Reporting. | |
Record name | C.I. Reactive Orange 4 | |
Source | EPA DSSTox | |
URL | https://comptox.epa.gov/dashboard/DTXSID4072079 | |
Description | DSSTox provides a high quality public chemistry resource for supporting improved predictive toxicology. | |
Record name | Trisodium 2-[[6-[(4,6-dichloro-1,3,5-triazin-2-yl)methylamino]-1-hydroxy-3-sulphonato-2-naphthyl]azo]naphthalene-1,5-disulphonate | |
Source | European Chemicals Agency (ECHA) | |
URL | https://echa.europa.eu/substance-information/-/substanceinfo/100.067.889 | |
Description | The European Chemicals Agency (ECHA) is an agency of the European Union which is the driving force among regulatory authorities in implementing the EU's groundbreaking chemicals legislation for the benefit of human health and the environment as well as for innovation and competitiveness. | |
Explanation | Use of the information, documents and data from the ECHA website is subject to the terms and conditions of this Legal Notice, and subject to other binding limitations provided for under applicable law, the information, documents and data made available on the ECHA website may be reproduced, distributed and/or used, totally or in part, for non-commercial purposes provided that ECHA is acknowledged as the source: "Source: European Chemicals Agency, http://echa.europa.eu/". Such acknowledgement must be included in each copy of the material. ECHA permits and encourages organisations and individuals to create links to the ECHA website under the following cumulative conditions: Links can only be made to webpages that provide a link to the Legal Notice page. | |
Record name | 1,5-Naphthalenedisulfonic acid, 2-[2-[6-[(4,6-dichloro-1,3,5-triazin-2-yl)methylamino]-1-hydroxy-3-sulfo-2-naphthalenyl]diazenyl]-, sodium salt (1:3) | |
Source | European Chemicals Agency (ECHA) | |
URL | https://echa.europa.eu/information-on-chemicals | |
Description | The European Chemicals Agency (ECHA) is an agency of the European Union which is the driving force among regulatory authorities in implementing the EU's groundbreaking chemicals legislation for the benefit of human health and the environment as well as for innovation and competitiveness. | |
Explanation | Use of the information, documents and data from the ECHA website is subject to the terms and conditions of this Legal Notice, and subject to other binding limitations provided for under applicable law, the information, documents and data made available on the ECHA website may be reproduced, distributed and/or used, totally or in part, for non-commercial purposes provided that ECHA is acknowledged as the source: "Source: European Chemicals Agency, http://echa.europa.eu/". Such acknowledgement must be included in each copy of the material. ECHA permits and encourages organisations and individuals to create links to the ECHA website under the following cumulative conditions: Links can only be made to webpages that provide a link to the Legal Notice page. | |
Retrosynthesis Analysis
AI-Powered Synthesis Planning: Our tool employs the Template_relevance Pistachio, Template_relevance Bkms_metabolic, Template_relevance Pistachio_ringbreaker, Template_relevance Reaxys, Template_relevance Reaxys_biocatalysis model, leveraging a vast database of chemical reactions to predict feasible synthetic routes.
One-Step Synthesis Focus: Specifically designed for one-step synthesis, it provides concise and direct routes for your target compounds, streamlining the synthesis process.
Accurate Predictions: Utilizing the extensive PISTACHIO, BKMS_METABOLIC, PISTACHIO_RINGBREAKER, REAXYS, REAXYS_BIOCATALYSIS database, our tool offers high-accuracy predictions, reflecting the latest in chemical research and data.
Strategy Settings
Precursor scoring | Relevance Heuristic |
---|---|
Min. plausibility | 0.01 |
Model | Template_relevance |
Template Set | Pistachio/Bkms_metabolic/Pistachio_ringbreaker/Reaxys/Reaxys_biocatalysis |
Top-N result to add to graph | 6 |
Feasible Synthetic Routes
Disclaimer and Information on In-Vitro Research Products
Please be aware that all articles and product information presented on BenchChem are intended solely for informational purposes. The products available for purchase on BenchChem are specifically designed for in-vitro studies, which are conducted outside of living organisms. In-vitro studies, derived from the Latin term "in glass," involve experiments performed in controlled laboratory settings using cells or tissues. It is important to note that these products are not categorized as medicines or drugs, and they have not received approval from the FDA for the prevention, treatment, or cure of any medical condition, ailment, or disease. We must emphasize that any form of bodily introduction of these products into humans or animals is strictly prohibited by law. It is essential to adhere to these guidelines to ensure compliance with legal and ethical standards in research and experimentation.