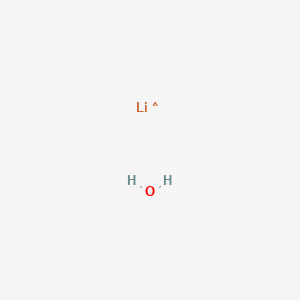
Lithium monohydrate
- Click on QUICK INQUIRY to receive a quote from our team of experts.
- With the quality product at a COMPETITIVE price, you can focus more on your research.
Overview
Description
Lithium hydroxide monohydrate (LiOH·H₂O) is an inorganic compound composed of lithium, oxygen, and hydrogen. Its structure, confirmed via X-ray and neutron diffraction, features strong ionic bonds between lithium and hydroxide ions, stabilized by hydrogen bonding with water molecules . Key properties include:
- High alkalinity: Acts as a strong base, reacting with CO₂ to form lithium carbonate (Li₂CO₃) and water, making it critical for CO₂ scrubbing in submarines and spacecraft .
- Thermal stability: Decomposes at elevated temperatures, releasing water and forming anhydrous LiOH, which further decomposes into Li₂O .
- Industrial applications: Used in lithium-ion battery cathodes (e.g., LiNiMnCoO₂), lubricants, pharmaceuticals, and corrosion control in nuclear reactors .
Classified into grades (e.g., LiOH·H₂O-D1, D2, D3) based on purity and particle size, battery-grade LiOH·H₂O requires ≤0.01% impurities like Fe and Ca .
Scientific Research Applications
Energy Storage
Lithium-ion Batteries:
Lithium hydroxide monohydrate is crucial in the production of high-performance lithium-ion batteries, especially those using nickel-rich cathodes such as NMC811 and NMC622. The compound is used to synthesize lithium nickel manganese cobalt oxide (NMC), which enhances battery efficiency and capacity. The demand for lithium hydroxide monohydrate in battery manufacturing is expected to rise significantly due to the increasing adoption of electric vehicles and renewable energy storage solutions .
Table 1: Comparison of Lithium Hydroxide Monohydrate in Battery Applications
Application Type | Description | Benefits |
---|---|---|
Cathode Material | Used in NMC batteries | Higher energy density, improved stability |
Electrolyte | Component in lithium-ion battery electrolytes | Enhances ionic conductivity |
Recycling | Used in recycling processes for lithium-ion batteries | Reduces environmental impact |
Environmental Applications
Carbon Dioxide Capture:
Recent studies have demonstrated the potential of lithium hydroxide monohydrate as an effective adsorbent for carbon dioxide capture. Its unique properties allow it to form stable complexes with CO₂, making it suitable for applications in air purification systems and carbon capture technologies. The low heat of reaction with CO₂ compared to other hydroxides enhances its efficiency in various environmental conditions .
Case Study: CO₂ Adsorption Efficiency
In a controlled experiment, modified zeolite adsorbents incorporating lithium hydroxide showed a significant increase in CO₂ adsorption capacity compared to unmodified versions. This suggests that lithium hydroxide can enhance the performance of existing materials used for carbon capture .
Industrial Applications
Lubricants and Greases:
Lithium hydroxide monohydrate is widely used in the formulation of lubricating greases. It acts as a thickening agent, providing excellent mechanical stability and resistance to water washout. Lithium-based greases are preferred in high-temperature applications due to their superior performance characteristics .
Table 2: Industrial Uses of Lithium Hydroxide Monohydrate
Industry | Application | Key Features |
---|---|---|
Lubricants | Thickening agent in greases | High thermal stability |
Ceramics | Component in ceramic production | Enhances durability |
Air Purification | CO₂ adsorbent for air quality improvement | Effective at ambient conditions |
Health and Safety Considerations
While lithium hydroxide monohydrate has numerous beneficial applications, it is essential to consider its health impacts. Exposure can lead to respiratory issues and other health complications if not handled properly. Safety assessments indicate that appropriate protective measures should be implemented during its use .
Chemical Reactions Analysis
Carbonation with Atmospheric CO₂
LiOH·H₂O reacts spontaneously with CO₂ to form lithium carbonate (Li₂CO₃) and water:
2LiOH⋅H2O+CO2→Li2CO3+3H2O
Key Findings:
-
Mechanism : The reaction proceeds via thermal dehydration of LiOH·H₂O to anhydrous LiOH, followed by CO₂ uptake. The process is geometrically controlled by the shrinkage of the reaction interface (LiOH·H₂O → LiOH → Li₂CO₃) .
-
Kinetics :
-
Thermodynamics : The reaction is exothermic with a negative Gibbs free energy change (ΔG°), confirming spontaneity .
Parameter | Value/Observation | Source |
---|---|---|
CO₂ Uptake Capacity | 450 mL CO₂ per 1 g LiOH·H₂O | |
Activation Energy | 80–120 kJ/mol (varies with CO₂ concentration) |
Thermal Decomposition
At elevated temperatures, LiOH·H₂O undergoes dehydration and decomposition:
2LiOH⋅H2OΔLi2O+3H2O(>600°C, inert atmosphere)
Key Findings:
-
Dehydration begins at ~100°C, forming anhydrous LiOH, which decomposes to Li₂O above 600°C .
-
Thermal stability is influenced by atmospheric CO₂, which promotes carbonation over decomposition .
Reactions with Acids
LiOH·H₂O neutralizes acids to form lithium salts:
Acid | Reaction Equation | Product |
---|---|---|
Sulfuric Acid | 2LiOH+H2SO4→Li2SO4+2H2O | Lithium Sulfate |
Phosphoric Acid | 3LiOH+H3PO4→Li3PO4+3H2O | Lithium Phosphate |
Hydrochloric Acid | LiOH+HCl→LiCl+H2O | Lithium Chloride |
Reactions with Metals
LiOH·H₂O reacts with amphoteric metals (e.g., Al, Zn) and alkaline earth metals (e.g., Mg, Ca):
With Magnesium:
2LiOH+Mg→2Li+MgO+H2O
With Zinc:
2LiOH+Zn→LiZnO2+H2
Reactions with Halogens
LiOH·H₂O reacts with halogens to form mixed lithium halides:
Halogen | Reaction Equation | Products |
---|---|---|
Cl₂ | 2LiOH+Cl2→LiCl+LiOCl+H2O | Lithium Chloride + Hypochlorite |
I₂ | 2LiOH+I2→LiI+LiOI+H2O | Lithium Iodide + Iodite |
Interaction with Water and Atmospheric Moisture
Q & A
Basic Research Questions
Q. What analytical methods are recommended for quantifying lithium hydroxide monohydrate purity in academic research?
Lithium hydroxide monohydrate purity is commonly assessed using acid-base titration (GB/T 11064.1-2013) for lithium carbonate content and potentiometric methods for chloride quantification (GB/T 11064.3-2013). For sulfate impurities, the barium sulfate turbidity method (GB/T 11064.9-2013) is standardized, with detection limits as low as 0.005% .
Q. How should researchers safely handle lithium hydroxide monohydrate in laboratory settings?
Strict adherence to SDS guidelines is critical: use chemical-resistant gloves (nitrile), full-face respirators for dust control, and ensure mechanical ventilation. Immediate decontamination protocols for skin/eye exposure involve 15-minute rinsing with water. Storage must avoid moisture, heat, and incompatible materials (e.g., acids) .
Q. What standardized methods exist for synthesizing lithium hydroxide monohydrate from lithium ore or brine?
GB/T 8766-2013 outlines industrial-scale synthesis from lithium ore or brine, emphasizing crystallization control and impurity removal. Key steps include electrolysis of lithium chloride and subsequent hydration, with product classification based on chemical composition (e.g., LiOH·H₂O-T1 for high-purity grades) .
Q. How can sulfate content in lithium hydroxide monohydrate be determined experimentally?
Sulfate quantification follows GB/T 11064.9-2013 using barium sulfate turbidimetry. The method involves acidifying the sample, adding barium chloride to precipitate sulfate ions, and measuring turbidity at 420 nm. Calibration curves are validated against certified reference materials .
Advanced Research Questions
Q. What advanced techniques are used for trace metal analysis in lithium hydroxide monohydrate?
Inductively coupled plasma atomic emission spectrometry (ICP-AES) (GB/T 11064.16-2023) enables simultaneous detection of 11+ impurities (e.g., Fe, Cu, Cd) at ppm levels. Spectral line selection (e.g., Ca II 317.933 nm) and matrix-matched calibration are critical to minimize interference .
Q. How can structural studies of lithium hydroxide monohydrate benefit from crystallographic software like SHELX?
SHELX programs (e.g., SHELXL for refinement) are optimized for high-resolution crystallography. For lithium hydroxide monohydrate, hydrogen-bonding networks and Li⁺ coordination geometry can be modeled using Fourier difference maps and anisotropic displacement parameters. ORTEP-3 aids in visualizing thermal ellipsoids and disorder .
Q. What methodologies address contradictions in analytical data between titration and spectroscopic techniques?
Cross-validation using complementary methods (e.g., titration for bulk LiOH and ICP-AES for trace metals) resolves discrepancies. Statistical analysis (e.g., Grubbs’ test) identifies outliers, while standard addition methods correct for matrix effects in complex samples .
Q. How does the choice of lithium source (brine vs. ore) impact life cycle greenhouse gas (GHG) emissions?
Argonne National Laboratory’s lifecycle analysis (2021) shows brine-derived lithium hydroxide monohydrate emits 25–30% less CO₂-equivalent than ore-based processes, primarily due to reduced energy demand in direct lithium extraction (DLE) from geothermal brines. However, ore processing allows tighter impurity control .
Q. What strategies optimize crystallization parameters for high-purity lithium hydroxide monohydrate?
Controlled cooling rates (0.5–1°C/min) and seed crystal introduction minimize inclusion of Na⁺/K⁺ impurities. Brine pre-treatment with ion-exchange resins (e.g., Li-selective sorbents) and pH stabilization at 12.5 enhance crystal uniformity .
Q. How can researchers assess chronic occupational exposure risks in lithium hydroxide monohydrate production facilities?
Air sampling (OSHA 1910.1020) combined with biomonitoring (urinary lithium levels) quantifies exposure. Engineering controls (closed-system processing) and PPE compliance reduce risks. Longitudinal studies track pulmonary edema incidence linked to particulate exposure .
Comparison with Similar Compounds
Comparison with Similar Lithium Compounds
Lithium Peroxide Monohydrate (Li₂O₂·H₂O)
- Structure and stability : Dehydrates at ~100°C to form Li₂O₂, which decomposes above 300°C, releasing oxygen (24.1% mass loss observed experimentally) .
- Applications : Oxygen generation systems due to its high active oxygen content (24.5% theoretical) .
Lithium Carbonate (Li₂CO₃)
- Production : LiOH·H₂O is a precursor to Li₂CO₃, which accounts for 71% of industrial lithium use due to simpler purification .
- Solubility : Li₂CO₃ has low water solubility (1.3 g/100 mL at 20°C), limiting its direct use in electrolytes, whereas LiOH·H₂O is highly soluble (12.8 g/100 mL) .
- Applications : Li₂CO₃ is a raw material for LiOH·H₂O and glass/ceramics, while LiOH·H₂O is preferred for batteries and greases .
Lithium Chloride Monohydrate (LiCl·H₂O) and Lithium Sulfate Monohydrate (Li₂SO₄·H₂O)
- Hydration and conductivity : LiCl·H₂O accelerates cement hydration by increasing Li⁺ ion concentration (0.05–0.22 mmol/g cement), while Li₂SO₄·H₂O enhances ionic conductivity in electrolytes .
- Crystal growth: Doped Li₂SO₄·H₂O exhibits nonlinear optical (NLO) properties, useful in laser technologies, unlike LiOH·H₂O’s role in energy storage .
Lithium Bromide (LiBr)
- Hygroscopicity : LiBr is highly hygroscopic, making it suitable for absorption chillers, whereas LiOH·H₂O’s CO₂ absorption is leveraged in air purification .
- Safety : LiBr is less corrosive than LiOH·H₂O, which requires careful handling due to its caustic nature .
Lithium Salts in Electrolytes (e.g., LiPF₆, LiAsF₆·H₂O)
Properties
CAS No. |
81408-53-9 |
---|---|
Molecular Formula |
H2LiO |
Molecular Weight |
25.0 g/mol |
InChI |
InChI=1S/Li.H2O/h;1H2 |
InChI Key |
FZRNJOXQNWVMIH-UHFFFAOYSA-N |
Canonical SMILES |
[Li].O |
Origin of Product |
United States |
Disclaimer and Information on In-Vitro Research Products
Please be aware that all articles and product information presented on BenchChem are intended solely for informational purposes. The products available for purchase on BenchChem are specifically designed for in-vitro studies, which are conducted outside of living organisms. In-vitro studies, derived from the Latin term "in glass," involve experiments performed in controlled laboratory settings using cells or tissues. It is important to note that these products are not categorized as medicines or drugs, and they have not received approval from the FDA for the prevention, treatment, or cure of any medical condition, ailment, or disease. We must emphasize that any form of bodily introduction of these products into humans or animals is strictly prohibited by law. It is essential to adhere to these guidelines to ensure compliance with legal and ethical standards in research and experimentation.