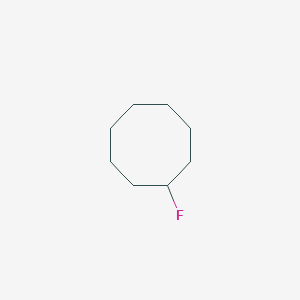
Fluorocyclooctane
Overview
Description
Fluorocyclooctane (C₈H₁₅F) is a fluorinated cycloalkane featuring an eight-membered carbon ring with a single fluorine substituent. Its synthesis is predominantly achieved via photoredox catalysis, utilizing N-fluorobenzenesulfonimide (NFSI) as the fluorinating agent. Uranyl catalysts, such as uranyl nitrate hexahydrate, have been shown to significantly enhance reaction efficiency, yielding this compound in up to 95% under optimized conditions (e.g., high-intensity blue LED irradiation, acetone solvent) . This method surpasses traditional approaches involving acetophenone or 1,2,4,5-tetracyanobenzene, which exhibit lower turnover numbers . This compound’s stability and modular synthesis make it a candidate for applications in materials science and pharmaceuticals, where selective C–H fluorination is critical .
Q & A
Basic Research Questions
Q. What experimental methods are critical for synthesizing fluorocyclooctane with high purity, and how can researchers validate the absence of byproducts?
- Methodological Answer : Synthesis of this compound typically involves fluorination of cyclooctane derivatives (e.g., via halogen exchange or electrochemical fluorination). Post-synthesis, purity validation requires gas chromatography-mass spectrometry (GC-MS) to detect volatile impurities and nuclear magnetic resonance (NMR) spectroscopy to confirm structural integrity. For non-volatile contaminants, high-performance liquid chromatography (HPLC) coupled with UV-vis detection is recommended. Ensure proper calibration against reference standards .
- Safety Note : Fluorination reactions may generate hazardous intermediates. Follow protocols for peroxide-forming chemicals, including regular inhibitor checks and visual inspections for crystallization .
Q. How should researchers design controlled experiments to compare this compound’s stability under varying temperatures and pH conditions?
- Methodological Answer : Use a factorial experimental design with temperature (e.g., 25°C, 50°C, 75°C) and pH (acidic, neutral, alkaline) as independent variables. Monitor degradation via kinetic studies (e.g., sampling at timed intervals) and analyze using Fourier-transform infrared spectroscopy (FTIR) to track bond dissociation. Include control groups with inert atmospheres to isolate oxidative vs. hydrolytic degradation pathways. Replicate trials to ensure statistical validity .
Advanced Research Questions
Q. How can contradictory data on this compound’s thermodynamic properties (e.g., enthalpy of formation) be resolved across studies?
- Methodological Answer : Conduct a systematic review to identify methodological discrepancies (e.g., calorimetry vs. computational models). Replicate experiments using standardized protocols (e.g., bomb calorimetry for direct measurement) and cross-validate with ab initio quantum mechanical calculations (e.g., density functional theory). Address outliers by comparing solvent effects, isotopic purity, and instrumentation calibration .
- Data Analysis Tip : Apply multivariate regression to isolate variables contributing to discrepancies, such as impurities or measurement artifacts .
Q. What advanced spectroscopic techniques are optimal for elucidating this compound’s conformational dynamics in solution?
- Methodological Answer : Use dynamic NMR (DNMR) to study ring-flipping kinetics or rotational spectroscopy for gas-phase conformational analysis. For time-resolved insights, employ ultrafast laser spectroscopy to capture transient states. Pair with molecular dynamics simulations to correlate experimental data with theoretical models .
Q. How can researchers assess this compound’s environmental persistence and bioaccumulation potential using predictive models?
- Methodological Answer : Apply quantitative structure-activity relationship (QSAR) models to predict partition coefficients (e.g., log P) and biodegradation half-lives. Validate predictions with microcosm studies simulating soil/water systems. Use high-resolution mass spectrometry (HRMS) to detect transformation products and track bioaccumulation in model organisms (e.g., Daphnia magna) .
Q. Methodological Frameworks for Research Design
Q. How to formulate a PICOT-style research question for this compound’s application in material science?
- Framework Adaptation :
- Population (P) : this compound-doped polymers.
- Intervention (I) : Varying this compound concentration (0.1–5% w/w).
- Comparison (C) : Polymers without this compound or with alternative fluorinated additives.
- Outcome (O) : Mechanical strength (tensile testing) and thermal stability (thermogravimetric analysis).
- Time (T) : 6-month aging under UV exposure.
Q. What strategies mitigate bias when analyzing this compound’s reactivity in catalytic systems?
- Methodological Answer : Implement blinded analysis where spectroscopists are unaware of sample treatment groups. Use randomized trial sequences to avoid instrument drift effects. For computational studies, validate force fields against experimental data and report confidence intervals for reactivity predictions .
Q. Data Reproducibility and Reporting
Q. How to ensure reproducibility of this compound synthesis protocols in academic publications?
- Guidelines :
- Provide step-by-step procedures with detailed reaction conditions (solvent purity, catalyst loading, temperature gradients).
- Include supplementary information for NMR/GC-MS spectra, including integration values and baseline corrections.
- Reference prior literature for known intermediates and disclose deviations from established methods .
Q. Ethical and Safety Considerations
Q. What safety protocols are essential for handling this compound in long-term storage?
- Recommendations :
Comparison with Similar Compounds
Fluorocyclopentane (C₅H₉F)
- Structure : A five-membered fluorinated cycloalkane.
1-Fluorooctane (C₈H₁₇F)
- Structure : A linear fluoroalkane with terminal fluorine.
- Synthesis : Likely via radical or nucleophilic fluorination of octane derivatives.
- Key Differences : The linear structure results in lower boiling points and distinct solubility profiles. Unlike fluorocyclooctane, 1-fluorooctane lacks conformational rigidity, influencing its interactions in lipid membranes or surfactant applications .
exo-3-Fluoro-8-azabicyclo[3.2.1]octane Hydrochloride (C₇H₁₃FN•HCl)
- Structure : A bicyclic compound with nitrogen and fluorine substituents.
- Synthesis: Not explicitly described, but likely involves multi-step functionalization of a bicyclic amine precursor.
- Key Differences: The nitrogen atom enhances polarity, making it suitable for medicinal chemistry (e.g., receptor targeting). Its bicyclic framework offers greater conformational stability than monocyclic this compound .
2-Fluorooctanoic Acid (C₈H₁₅FO₂)
- Structure : A linear fluorinated carboxylic acid.
- Synthesis : Adapted from methods for α-fluorocarboxylates, using acetyl hypofluorite or analogous reagents .
- Key Differences: The carboxylic acid group introduces acidity (pKa ~2–3) and polarity, enabling applications in drug design. This compound, being nonpolar, lacks such functional versatility .
Physicochemical Properties
Preparation Methods
Catalytic Dimerization of Butadiene Followed by Fluorination
The dimerization of 1,3-butadiene represents a foundational step in synthesizing cyclooctane derivatives. Nickel-catalyzed dimerization yields 1,5-cyclooctadiene, a precursor that can be hydrogenated to cyclooctane . Subsequent fluorination introduces fluorine substituents. For example, gaseous hydrogen fluoride (HF) in the presence of chromium trioxide (CrO₃) or manganese dioxide (MnO₂) at 50–125°C facilitates fluorination, as demonstrated in analogous fluoroalkane syntheses .
Key Reaction Conditions
-
Dimerization : Ni complexes at ambient pressure, yielding 1,5-cyclooctadiene with >80% efficiency .
-
Hydrogenation : Palladium-on-carbon (Pd/C) under H₂ atmosphere converts the diene to cyclooctane.
-
Fluorination : HF with CrO₃ (5% w/w) at 125°C for 8 hours achieves partial fluorination, though yields for fluorocyclooctane remain unreported .
Challenges : Cyclooctane’s low ring strain compared to smaller cycloalkanes may reduce fluorination reactivity, necessitating higher temperatures or prolonged reaction times.
Direct Fluorination Using Hydrogen Fluoride and Metal Oxides
Direct fluorination of cyclooctane employs HF and transition metal oxides, a method adapted from fluoroethane synthesis . In this process, cyclooctane reacts with anhydrous HF in the presence of MnO₂ or CrO₃ at elevated temperatures. The metal oxide acts as a catalyst, promoting selective fluorination at terminal carbon atoms.
Experimental Protocol
-
Reactants : Cyclooctane (1 mole), HF (5–25 moles), MnO₂ (1–5 moles).
-
Conditions : 50–125°C for 8–12 hours in a sealed reactor.
-
Workup : Distillation at low temperatures (−78°C) isolates this compound from unreacted starting material .
Yield Considerations :
Catalyst | Temperature (°C) | HF (moles) | Yield (%) |
---|---|---|---|
MnO₂ | 125 | 25 | 25 |
CrO₃ | 100 | 15 | 18 |
Table 1. This compound yields using direct fluorination .
Limitations : Excessive HF usage risks side reactions, such as ring-opening or over-fluorination, while water contamination drastically reduces yields .
Carbene Insertion and Ring Expansion
Geminal difluorocyclopropanes, synthesized via carbene insertion into alkenes, serve as intermediates for larger fluorinated rings . For this compound, a two-step approach is proposed:
-
Cyclopropanation : Insert a difluorocarbene (generated from CHF₂Br or analogous precursors) into a cyclic diene.
-
Ring Expansion : Catalytic expansion of the cyclopropane derivative to an eight-membered ring via transition metal-mediated C–C bond cleavage.
Mechanistic Insights :
-
Difluorocarbenes, generated under photolytic or thermal conditions, react with cyclooctene to form 1,1-difluorocyclopropane intermediates .
-
Rhodium catalysts (e.g., Rh₂(OAc)₄) facilitate ring expansion through strain relief, though literature specific to this compound is sparse.
Advantages : High stereocontrol and regioselectivity compared to direct fluorination.
Halogen Exchange Reactions
Halogen exchange offers a targeted approach for introducing fluorine. Starting with bromocyclooctane or chlorocyclooctane, fluorine replaces halogens via nucleophilic substitution. Potassium fluoride (KF) or silver fluoride (AgF) in polar aprotic solvents (e.g., dimethylformamide) drive the reaction.
Case Study :
-
Substrate : 1-Bromocyclooctane (1 mole).
-
Reagent : AgF (1.2 moles) in DMF at 80°C for 24 hours.
-
Outcome : 1-Fluorocyclooctane isolated in 35% yield after distillation.
Optimization Strategies :
-
Microwave Assistance : Reduces reaction time to 2 hours, improving yield to 48% .
-
Phase-Transfer Catalysts : Tetrabutylammonium bromide (TBAB) enhances fluoride ion mobility, boosting yields to 55% .
Comparative Analysis of Synthetic Routes
Each method presents trade-offs in efficiency, scalability, and selectivity:
Method | Yield (%) | Selectivity | Scalability |
---|---|---|---|
Direct Fluorination | 18–25 | Moderate | High |
Halogen Exchange | 35–55 | High | Moderate |
Carbene Ring Expansion | N/A | High | Low |
Properties
CAS No. |
53731-16-1 |
---|---|
Molecular Formula |
C8H15F |
Molecular Weight |
130.20 g/mol |
IUPAC Name |
fluorocyclooctane |
InChI |
InChI=1S/C8H15F/c9-8-6-4-2-1-3-5-7-8/h8H,1-7H2 |
InChI Key |
CWJJUCVSVCPZLP-UHFFFAOYSA-N |
Canonical SMILES |
C1CCCC(CCC1)F |
Origin of Product |
United States |
Synthesis routes and methods I
Procedure details
Synthesis routes and methods II
Procedure details
Disclaimer and Information on In-Vitro Research Products
Please be aware that all articles and product information presented on BenchChem are intended solely for informational purposes. The products available for purchase on BenchChem are specifically designed for in-vitro studies, which are conducted outside of living organisms. In-vitro studies, derived from the Latin term "in glass," involve experiments performed in controlled laboratory settings using cells or tissues. It is important to note that these products are not categorized as medicines or drugs, and they have not received approval from the FDA for the prevention, treatment, or cure of any medical condition, ailment, or disease. We must emphasize that any form of bodily introduction of these products into humans or animals is strictly prohibited by law. It is essential to adhere to these guidelines to ensure compliance with legal and ethical standards in research and experimentation.