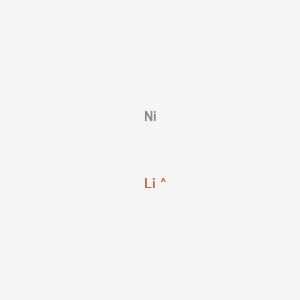
Lithium nickel
- Click on QUICK INQUIRY to receive a quote from our team of experts.
- With the quality product at a COMPETITIVE price, you can focus more on your research.
Description
Lithium nickel-based compounds, such as this compound dioxide (LiNiO₂) and its derivatives (e.g., NMC: LiNiₓMnᵧCo₂O₂), are critical cathode materials in rechargeable lithium-ion batteries (LIBs). These compounds are characterized by their layered oxide structures, which facilitate lithium-ion intercalation and deintercalation during charge/discharge cycles .
Key Properties of this compound Dioxide (LiNiO₂):
- Chemical Structure: Layered O3-type structure with alternating Li and NiO₂ layers .
- Thermal Stability: Delithiated LiₓNiO₂ (e.g., Li₀.₂NiO₂) exhibits exothermic reactions with electrolytes, releasing oxygen and posing safety risks at elevated temperatures .
- Electrochemical Performance: High theoretical capacity (~274 mAh/g) but suffers from structural instability due to nickel migration into lithium vacancies during cycling .
This compound compounds are often modified with cobalt, manganese, or aluminum (e.g., NMC, NCA) to enhance stability and capacity retention. These modifications address inherent challenges such as cation mixing and oxygen release .
Q & A
Basic Research Questions
Q. What established synthesis methods are used for lithium nickel oxide cathode materials, and how do they influence electrochemical performance?
this compound oxide (LiNiO₂) is synthesized via high-temperature solid-state reactions (>700°C), sol-gel methods, or co-precipitation. Solid-state methods yield layered structures but risk cation mixing, while sol-gel techniques improve particle homogeneity. Key parameters include calcination temperature and precursor ratios to stabilize Ni³+ and minimize structural degradation during cycling .
Q. How can researchers assess the environmental impacts of nickel and lithium extraction in battery supply chains?
Life cycle assessments (LCAs) should integrate water consumption (e.g., brine vs. hard-rock mining), energy use, and GHG emissions. For lithium brines, monitor groundwater depletion via piezometric sensors. For nickel laterites, analyze tailing management and soil toxicity. Comparative studies must include site-specific biodiversity metrics and long-term ecological monitoring .
Q. What experimental protocols are recommended for evaluating the electrochemical stability of this compound-based batteries?
Cyclic voltammetry (CV) at 0.1–1 mV/s scan rates identifies redox peaks, while galvanostatic cycling (0.5–2C rates) assesses capacity retention. Post-cycling analysis via XRD detects phase transitions, and SEM-EDS maps surface degradation. Electrolyte compatibility tests (e.g., GC-MS for decomposition products) are critical for stability assessments .
Q. How should thermal management systems for this compound batteries be experimentally validated?
Use nickel foam-paraffin composites as phase-change materials (PCMs) in adiabatic chambers. Measure heat dissipation rates using thermocouples and infrared imaging. Validate models against experimental data to optimize PCM thickness and porosity for thermal runaway mitigation .
Q. What are the best practices for reporting experimental data on this compound compounds in academic publications?
Follow guidelines for detailed methodology sections: include synthesis parameters (precursors, temperatures), characterization techniques (XRD, TEM), and statistical validation (e.g., error margins for electrochemical measurements). Use supplementary materials for extensive datasets, and reference protocols from authoritative journals .
Advanced Research Questions
Q. How can researchers resolve discrepancies in nickel content measurements across electrochemical systems, such as molten carbonate fuel cells?
Cross-validate using neutron diffraction (bulk Ni distribution) and XPS (surface analysis). Standardize post-test matrix digestion protocols and ICP-MS calibration. Differences in Li-rich vs. K-rich electrolytes require controlled experiments to isolate dissolution effects .
Q. What advanced in situ characterization techniques are critical for studying phase transitions in high-nickel NCM cathodes during fast charging?
Operando XRD tracks lattice dynamics, while synchrotron XAS at Ni K-edge monitors oxidation states. Pair with EIS to decouple interfacial resistance contributions. Cryo-TEM can capture metastable phases and Li/Ni antisite defects .
Q. What novel hydrometallurgical methods improve recovery efficiency of nickel and lithium from spent batteries?
Combine organic acids (e.g., citric acid) with redox mediators to selectively leach Ni and Li at <100°C. Use solvent extraction (e.g., D2EHPA for Ni) and electrowinning for high-purity metal recovery. Compare with bioleaching (e.g., Acidithiobacillus spp.) for sustainable scaling .
Q. How should adiabatic calorimetry experiments be designed to assess thermal runaway risks in ultra-high nickel NCM batteries?
Use hermetically sealed calorimeters (e.g., BAC-420A) with high-speed thermocouples (≥100 Hz) and pressure sensors. Pre-condition cells at 100% SOC, and analyze gas emissions via mass spectrometry. Report time-to-thermal-runaway (TTR) and self-heating rates (dT/dt) for failure modeling .
Q. What computational models are effective for predicting degradation mechanisms in this compound manganese oxide (LNMO) cathodes?
Density functional theory (DFT) simulations identify oxygen vacancy formation energies, while finite element analysis (FEA) models stress distributions during lithiation. Validate with operando Raman spectroscopy to correlate mechanical strain with capacity fade .
Q. Methodological Resources
- Data Reporting : Include raw electrochemical datasets (charge/discharge curves, EIS spectra) in supplementary files. Use standardized formats (e.g., .csv) for reproducibility .
- Environmental Metrics : Adopt ISO 14040/44 frameworks for LCAs, with region-specific water stress indices from the World Resources Institute (WRI) .
- Safety Testing : Follow UL 1642 protocols for thermal abuse tests, and document failure modes using high-speed video and gas chromatography .
Comparison with Similar Compounds
Comparison with Similar Cathode Materials
Energy Density and Capacity
Key Findings :
- LiNiO₂ and NMC variants outperform LFP in energy density, making them preferable for electric vehicles (EVs) requiring long range .
- However, NMC and LiNiO₂ require cobalt or manganese to mitigate nickel’s structural instability, increasing material costs .
Thermal and Chemical Stability
- LiNiO₂ : Generates significant exothermic heat (~500 J/g) when delithiated (Li₀.₂NiO₂) and exposed to ethylene carbonate solvents, primarily due to oxygen release .
- NMC : Partial substitution of nickel with manganese reduces oxygen release, lowering thermal runaway risk compared to LiNiO₂ .
Environmental and Economic Impact
Ecological Footprint (Emissions in m²a) :
Material | Emissions (m²a) |
---|---|
Standard LIB (LiCoO₂) | 9,019.91 |
NMC | 6,061.29 |
LFP | 5,049.44 |
Cost and Resource Availability :
- Cobalt Dependency : LCO and NMC face supply chain risks due to cobalt’s scarcity and ethical mining concerns. NMC-532 (Ni:Mn:Co = 5:3:2) reduces cobalt use by 40% compared to LCO .
- Nickel Abundance : Nickel’s crustal abundance (70 ppm) matches lithium, making it a sustainable alternative if structural challenges are addressed .
Cycle Life and Rate Capability
- LiNiO₂ : Rapid capacity fade (>20% after 500 cycles) due to nickel migration .
- NMC-811 : Achieves 80% capacity retention after 1,000 cycles with optimized nickel content .
- Fe-doped Ni(OH)₂ : Enhances Li⁺ diffusivity by 30% and reduces electrochemical resistance compared to pure nickel hydroxide .
Improvement Strategies :
- Surface coatings (e.g., Al₂O₃) and dopants (e.g., Fe, Al) mitigate degradation in nickel-rich cathodes .
Critical Challenges :
- Thermal management for nickel-rich cathodes.
- Recycling complexities due to mixed metal compositions .
Properties
CAS No. |
67907-41-9 |
---|---|
Molecular Formula |
LiNi |
Molecular Weight |
65.7 g/mol |
IUPAC Name |
lithium;nickel |
InChI |
InChI=1S/Li.Ni |
InChI Key |
RSNHXDVSISOZOB-UHFFFAOYSA-N |
Canonical SMILES |
[Li].[Ni] |
Origin of Product |
United States |
Disclaimer and Information on In-Vitro Research Products
Please be aware that all articles and product information presented on BenchChem are intended solely for informational purposes. The products available for purchase on BenchChem are specifically designed for in-vitro studies, which are conducted outside of living organisms. In-vitro studies, derived from the Latin term "in glass," involve experiments performed in controlled laboratory settings using cells or tissues. It is important to note that these products are not categorized as medicines or drugs, and they have not received approval from the FDA for the prevention, treatment, or cure of any medical condition, ailment, or disease. We must emphasize that any form of bodily introduction of these products into humans or animals is strictly prohibited by law. It is essential to adhere to these guidelines to ensure compliance with legal and ethical standards in research and experimentation.