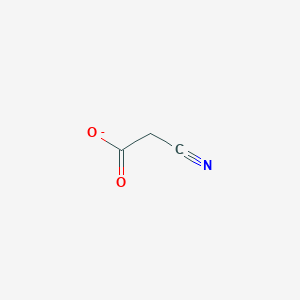
Cyanoacetate
- Click on QUICK INQUIRY to receive a quote from our team of experts.
- With the quality product at a COMPETITIVE price, you can focus more on your research.
Overview
Description
Cyanoacetate, specifically ethyl this compound (ECA; C₅H₷NO₂), is a versatile organic compound widely used as a key intermediate in pharmaceuticals, agrochemicals, and specialty chemicals. Its synthesis typically involves the reaction of chloroacetic acid with sodium cyanide, followed by esterification with ethanol . ECA’s α-cyanoester structure enables diverse reactivity, such as Knoevenagel condensations, cyclizations, and nucleophilic substitutions, making it indispensable in constructing heterocycles (e.g., benzothiazoles, pyrimidines) and functionalized molecules . The global ethyl this compound market is projected to grow at a CAGR of 4.9%, driven by demand in drug synthesis and agrochemical production .
Scientific Research Applications
Chemical Synthesis
1.1 Building Block for Organic Compounds
Cyanoacetate serves as an essential intermediate in organic synthesis. Its functional groups allow it to participate in various chemical reactions, making it a valuable precursor for synthesizing complex molecules. Notably, ethyl this compound is utilized to create heterocycles, which are crucial components in many pharmaceutical agents . The compound can undergo reactions such as:
- Nucleophilic attacks at the nitrile and ester groups.
- Hydrogenation , leading to the formation of β-amino acids like β-alanine.
- Condensation reactions , which are fundamental for creating more complex structures .
1.2 Synthesis of Heterocycles
Heterocycles synthesized from this compound are integral to many drugs. For instance, the compound is involved in the production of various pharmaceutical agents, including dextromethorphan (a cough suppressant), amiloride (a diuretic), and allopurinol (used for gout treatment) . The ability to modify this compound through various synthetic pathways enhances its utility in drug development.
Pharmaceutical Applications
2.1 Drug Development
This compound is extensively used in the pharmaceutical industry due to its ability to act as a precursor for several active pharmaceutical ingredients (APIs). It plays a pivotal role in synthesizing compounds that target various medical conditions, including:
- Anticancer agents : this compound derivatives are used to develop drugs that inhibit cancer cell proliferation.
- Vitamins and steroids : The compound is involved in synthesizing essential vitamins and steroid hormones .
The growing demand for innovative pharmaceuticals drives the market for this compound derivatives, as researchers continue to explore new therapeutic compounds .
2.2 Case Studies
Recent studies have demonstrated the effectiveness of using this compound in synthesizing novel compounds with enhanced pharmacological properties. For example, research into the salen(Al)-catalyzed enantioselective Michael addition of methyl this compound has shown promising results in achieving high stereoselectivity, which is crucial for drug efficacy .
Industrial Applications
3.1 Adhesives and Sealants
Cyanoacrylate adhesives, derived from this compound compounds, are widely used across various industries due to their fast-setting properties and strong bonding capabilities. These adhesives find applications in:
- Automotive manufacturing : Used for securing components and repairing parts.
- Medical applications : Employed for wound closure and surgical procedures due to their biocompatibility .
3.2 Dyes and Pigments
This compound also serves as an intermediate in producing dyes and pigments, such as malachite green and phthalocyanine pigments. These colorants are utilized in textiles, printing inks, and paints, showcasing the compound's versatility beyond pharmaceuticals .
Chemical Reactions Analysis
Knoevenagel Condensation
Ethyl cyanoacetate undergoes condensation with aldehydes to form α,β-unsaturated nitriles. This reaction is widely used in heterocycle and pharmaceutical synthesis.
Catalysts and Conditions:
Mechanism :
-
Deprotonation of the acidic methylene group forms a nucleophilic enolate.
-
The enolate attacks the aldehyde carbonyl, followed by dehydration to yield the α,β-unsaturated product .
Applications :
Michael Addition
The methylene group acts as a nucleophile in conjugate additions to α,β-unsaturated carbonyl compounds.
Example :
-
Reaction with methyl acrylate forms β-cyano-β-ethoxycarbonyl derivatives, intermediates for β-amino acids .
Palladium-Catalyzed Arylation
Ethyl this compound undergoes α-arylation with aryl halides under Pd catalysis.
Substrate Scope (Selected Examples):
Aryl Halide | Ligand | Yield (%) | Reference |
---|---|---|---|
4-Bromotoluene | P(o-Tol)₃ | 89 | |
2-Chloronaphthalene | DavePhos | 78 | |
3,5-Dimethoxybromobenzene | XantPhos | 82 |
Conditions :
Polymerization
This compound derivatives (e.g., methyl cyanoacrylate) polymerize via anionic mechanisms.
Mechanism :
-
Initiation : Nucleophilic attack by water/OH⁻ on the electrophilic β-carbon.
-
Propagation : Sequential addition of monomers to the growing chain.
Thermodynamics :
Hydrogenation
The nitrile group is reduced to an amine, forming β-alanine derivatives.
Conditions :
Ester Hydrolysis
Hydrolysis rates depend on pH:
Condition | Half-Life (25°C) | Product | Source |
---|---|---|---|
Neutral pH | 2 hours | Cyanoacetic acid | |
Alkaline pH | <30 minutes | Cyanoacetic salt | |
Acidic pH | >24 hours | Stable ester |
Sequential Allylic Alkylation
Iridium-catalyzed double allylic alkylation forms stereochemically complex products.
Stereochemical Outcomes:
Allylic Carbonate 1 | Allylic Carbonate 2 | Configuration (Major Product) | ee (%) | dr | Source |
---|---|---|---|---|---|
4-Bromo-cinnamyl | 2-Naphthyl | (S,S,S) | 99 | 20:1 | |
3,4-Dimethoxybenzyl | 1-Cyclohexenyl | (R,R,R) | 98 | 15:1 |
Mechanism :
Nitrile Functionalization
Reactions at the nitrile group include:
Q & A
Basic Research Questions
Q. What are the primary safety considerations when handling cyanoacetate derivatives in laboratory settings?
Methodological Answer:
- Always use personal protective equipment (PPE), including chemical-resistant gloves (JIS T 8116 standard), protective eyewear, and long-sleeved clothing, to prevent skin/eye contact .
- Ensure local exhaust ventilation and seal reaction sources to minimize inhalation risks .
- In case of accidental exposure, follow first-aid protocols: flush eyes/skin with water for 15+ minutes, and seek immediate medical attention .
- Store cyanoacetates in tightly sealed containers away from heat to avoid toxic gas release during decomposition .
Q. What experimental parameters are critical for optimizing ethyl this compound synthesis via esterification?
Methodological Answer:
- Key factors include catalyst concentration (e.g., sulfuric acid), molar ratio of reactants (cyanoacetic acid to ethanol), temperature (optimal: 80°C), and reaction time (3.5 hours) .
- Use orthogonal experimental design to rank parameter influence. For example, catalyst amount has the greatest impact on esterification yield, followed by molar ratio and temperature .
- Validate optimal conditions using gas chromatography (GC) to quantify purity and yield (e.g., Figure 3 and Table 3 in ).
Q. How should researchers design a study to replicate published this compound synthesis protocols?
Methodological Answer:
- Include detailed experimental sections in manuscripts, specifying reagent purity, equipment calibration, and procedural nuances (e.g., stirring rate, inert gas use) .
- For known compounds, cross-validate identity via IR spectroscopy (e.g., ethyl this compound’s carbonyl and nitrile peaks at ~1740 cm⁻¹ and ~2250 cm⁻¹) .
- Provide raw data and chromatograms in supplementary materials to enable replication .
Advanced Research Questions
Q. How can contradictions in reported reaction mechanisms of this compound intermediates be resolved?
Methodological Answer:
- Conduct kinetic studies to identify rate-determining steps (e.g., nucleophilic vs. electrophilic pathways).
- Use isotopic labeling (e.g., ¹³C or ²H) to trace reaction pathways and intermediate stability .
- Compare results across solvents and catalysts to isolate mechanistic variables (e.g., solvent polarity effects on Knoevenagel condensation) .
Q. What advanced analytical techniques are recommended for characterizing this compound reaction pathways?
Methodological Answer:
- Gas Chromatography-Mass Spectrometry (GC-MS): Quantify intermediates and byproducts (e.g., ethyl this compound esterification byproducts) .
- In Situ IR Spectroscopy: Monitor real-time functional group changes (e.g., nitrile and ester peak shifts during reactions) .
- Computational Modeling: Use DFT calculations to predict transition states and validate experimental observations .
Q. How can researchers address discrepancies in this compound toxicity data across studies?
Methodological Answer:
- Standardize toxicity assays (e.g., acute oral toxicity in rodent models) and report LD₅₀ values with confidence intervals .
- Control for variables like compound purity, solvent residues, and exposure duration .
- Cross-reference regulatory databases (e.g., CAMEO Chemicals, NIST) for harmonized hazard classifications .
Q. What strategies improve the environmental safety of this compound waste disposal?
Methodological Answer:
- Neutralize waste with alkaline solutions (e.g., sodium bicarbonate) to hydrolyze nitrile groups into less toxic carboxylates .
- Use adsorption materials (e.g., activated carbon) to capture residual compounds before disposal .
- Adhere to JIS Z 7253:2019 standards for waste treatment and document compliance .
Q. Methodological Frameworks
Q. How can the PICOT framework be adapted to structure this compound-related research questions?
Methodological Answer:
- Population (P): Define the chemical system (e.g., this compound derivatives in organic synthesis).
- Intervention (I): Specify the variable (e.g., catalyst type, solvent).
- Comparison (C): Include control experiments (e.g., uncatalyzed reactions).
- Outcome (O): Measure success via yield, purity, or toxicity metrics.
- Time (T): Define reaction duration or long-term stability tests .
Q. What statistical methods are suitable for analyzing this compound experimental data?
Methodological Answer:
- Use ANOVA to compare yields across multiple reaction conditions .
- Apply principal component analysis (PCA) to identify correlations between variables (e.g., temperature vs. byproduct formation) .
- Report uncertainties using standard deviation or confidence intervals for reproducibility .
Q. How to identify research gaps in this compound applications using literature review tools?
Methodological Answer:
- Search Google Scholar with keywords (e.g., "this compound catalysis") and filter by citation count to prioritize influential studies .
- Use SciFinder to map reaction pathways and identify understudied intermediates (e.g., this compound-metal complexes) .
- Analyze review articles in Advanced Synthesis & Catalysis for trends and unresolved challenges .
Q. Tables for Quick Reference
Table 1: Optimal Conditions for Ethyl this compound Synthesis
Parameter | Optimal Value |
---|---|
Molar Ratio (Acid:EtOH) | 1:3.5 |
Catalyst Concentration | 1.5% (w/w) |
Temperature | 80°C |
Reaction Time | 3.5 hours |
Table 2: Key IR Peaks for Ethyl this compound
Functional Group | Wavenumber (cm⁻¹) |
---|---|
C≡N (Nitrile) | ~2250 |
C=O (Ester) | ~1740 |
Comparison with Similar Compounds
Methyl Cyanoacetate (MCA; C₄H₅NO₂)
Synthesis: MCA is synthesized similarly to ECA but substitutes ethanol with methanol. It is also produced via Knoevenagel condensation under solvent-free conditions using calcite or fluorite catalysts . Applications:
- Serves as a glyoxylate anion synthon in 1,2,5-tricarbonyl compound synthesis .
- Used in pyrazole synthesis via hydrazide condensations . Reactivity: Compared to ECA, MCA exhibits slightly lower steric hindrance, enhancing its efficiency in nucleophilic additions. However, its shorter ethyl chain reduces solubility in nonpolar solvents.
Isobutyl this compound (IBCA; C₇H₁₁NO₂)
Synthesis: IBCA replaces ethanol with isobutanol during esterification. Applications: Primarily used in specialty polymers and flavor/fragrance industries due to its branched alkyl chain, which improves thermal stability . Reactivity: The bulky isobutyl group increases steric hindrance, slowing reaction kinetics in cyclocondensations compared to ECA .
Sodium this compound (SCA; C₃H₂NNaO₂)
Synthesis: Prepared by neutralizing cyanoacetic acid with sodium hydroxide. Applications:
- Used in aqueous-phase reactions due to high water solubility .
- Acts as a carboxy nucleophile in F-TEDA-BF4-mediated benzylic functionalizations . Reactivity: Unlike ECA, SCA’s ionic nature limits its utility in nonpolar solvents but enhances its role in green chemistry applications.
Potassium this compound (PCA; C₃H₂KNO₂)
Reactivity : PCA exhibits dual nucleophilicity—its cyanide group reacts in trifluoroacetic acid (TFA), while the carboxylate group dominates in acetonitrile (MeCN) . This solvent-dependent behavior contrasts with ECA, which primarily undergoes ester-based reactions.
Research Findings and Industrial Relevance
- Antitumor Activity: ECA-derived benzothiazolethienopyrimidines (e.g., compound 67) show high activity against cancer cell lines (IC₅₀: 0.8–2.1 µM) .
- Catalytic Efficiency : ECA’s compatibility with ionic liquid catalysts ([Ch][OH]) enables efficient heterocycle synthesis (yields: 75–92%) .
- Sustainability : ECA-based branched fluorous tags minimize reliance on expensive perfluorohexylpropyl iodide, reducing production costs by ~30% .
- Safety : While ECA derivatives are generally safe, sodium chloroacetate (a precursor) requires stringent handling due to acute toxicity (LD₅₀: 150 mg/kg in rats) .
Preparation Methods
Nucleophilic Substitution Using Chloroacetate and Sodium Cyanide
Reaction Mechanism and Process Design
This method involves the displacement of chloride in chloroacetate by cyanide ions under alkaline conditions, forming cyanoacetate and sodium chloride as a byproduct. The continuous-flow system described in patent CN102633682A utilizes a pipeline reactor for rapid mixing and controlled residence times, minimizing side reactions . Chloroacetate (e.g., methyl chloroacetate) and sodium cyanide are dissolved in alcoholic solvents (methanol, ethanol, or butanol) at concentrations of 10–90% and 10–20%, respectively. A molar ratio of 1:0.9–1.1 (chloroacetate:sodium cyanide) is maintained, with organic monoacids (e.g., acetic acid) adjusting the pH to 5–8 to suppress hydrolysis .
Optimization and Scalability
Key parameters include:
-
Temperature : 60–150°C (preheating at 60–120°C, reaction at 65–150°C)
-
Pressure : 0.05–1.0 MPa
-
Residence time : 4–10 minutes
-
Solvent flow rates : 500–3000 L/h
As shown in Table 1, varying the alcohol solvent and chloroacetate structure impacts yield and purity. For example, butyl chloroacetate in butanol achieves 86.3% yield at 99.55% purity, while isopropyl chloroacetate in isopropanol yields 83.0% . The continuous process eliminates batch-mode inefficiencies, enabling 24-hour production cycles with >99.5% purity in industrial settings .
Table 1: Performance of Continuous-Flow this compound Synthesis
Chloroacetate | Solvent | Sodium Cyanide Concentration | Molar Ratio | Yield (%) | Purity (%) |
---|---|---|---|---|---|
Methyl | Methanol | 10% | 1:1 | 84.3 | 99.70 |
Ethyl | Ethanol | 20% | 1:0.95 | 83.1 | 99.60 |
Butyl | Butanol | 15% | 1:0.9 | 86.3 | 99.55 |
Sulfuric Acid-Catalyzed Esterification of Cyanoacetic Acid
Traditional Esterification Protocol
Ethyl this compound is synthesized via acid-catalyzed esterification of cyanoacetic acid with ethanol. Patent CN109020836A details a process where cyanoacetic acid and ethanol (1:3–4 molar ratio) react with 98% sulfuric acid (0.08–0.2 mass ratio) at 55–85°C . Sulfur trioxide (SO₃) is introduced to accelerate dehydration, achieving 4-hour reaction times with subsequent distillation to recover ethanol .
Limitations and Modifications
While effective, this method faces challenges:
-
Corrosion : Concentrated sulfuric acid damages equipment.
-
Byproducts : Over-esterification and polymerization occur at elevated temperatures.
-
Workup complexity : Neutralization with ammonia water generates sulfate waste .
Mixed Acid-Catalyzed Esterification for Enhanced Efficiency
Catalyst Design and Orthogonal Optimization
A study by Yingkou Institute of Technology combined silicotungstic acid and p-toluene sulfonic acid (1:1 ratio) to catalyze cyanoacetic acid and ethanol esterification . Orthogonal experiments identified optimal conditions:
-
Catalyst loading : 1.5% of reactants
-
Molar ratio (acid:alcohol) : 1:4
-
Temperature : 80°C
-
Time : 5 hours
Gas chromatography confirmed esterification rates exceeding 90%, with the mixed catalyst enhancing proton availability and reducing side reactions .
Advantages Over Sulfuric Acid
-
Lower corrosivity : Reduced equipment degradation.
-
Higher selectivity : Minimal dimerization of cyanoacetic acid.
-
Reusability : Catalysts retain activity for 3–5 cycles after filtration .
Ionic Liquid-Mediated Green Synthesis
Sustainable Reaction Platforms
Patent CN102786436B introduces ionic liquids (ILs) as dual solvents and catalysts for this compound synthesis . Glyoxaline, pyrrolidinone, and pyridine-sulfonic acid ILs (e.g., [HSO₄⁻]-based variants) facilitate esterification at 40–100°C with 2–15-hour reaction times . For example, cyanoacetic acid and benzyl alcohol in [PSPy][HSO₄] yield 89% benzyl this compound after 8 hours at 80°C .
Environmental and Economic Benefits
-
Recyclability : ILs are recovered via simple phase separation, maintaining >95% activity after 5 cycles.
-
Waste reduction : No neutralization steps required, eliminating sulfate byproducts.
-
Broad substrate scope : Compatible with C₁–C₈ alcohols, cyclohexanol, and benzyl alcohol .
Table 2: Ionic Liquid Performance in this compound Synthesis
Ionic Liquid Type | Alcohol | Temperature (°C) | Time (h) | Yield (%) |
---|---|---|---|---|
[Py-SO₃H][HSO₄] | Ethanol | 70 | 6 | 91 |
[Bmim][HSO₄] | Cyclohexanol | 90 | 10 | 85 |
[Pyrr][HSO₄] | Benzyl | 80 | 8 | 89 |
Properties
Molecular Formula |
C3H2NO2- |
---|---|
Molecular Weight |
84.05 g/mol |
IUPAC Name |
2-cyanoacetate |
InChI |
InChI=1S/C3H3NO2/c4-2-1-3(5)6/h1H2,(H,5,6)/p-1 |
InChI Key |
MLIREBYILWEBDM-UHFFFAOYSA-M |
Canonical SMILES |
C(C#N)C(=O)[O-] |
Origin of Product |
United States |
Disclaimer and Information on In-Vitro Research Products
Please be aware that all articles and product information presented on BenchChem are intended solely for informational purposes. The products available for purchase on BenchChem are specifically designed for in-vitro studies, which are conducted outside of living organisms. In-vitro studies, derived from the Latin term "in glass," involve experiments performed in controlled laboratory settings using cells or tissues. It is important to note that these products are not categorized as medicines or drugs, and they have not received approval from the FDA for the prevention, treatment, or cure of any medical condition, ailment, or disease. We must emphasize that any form of bodily introduction of these products into humans or animals is strictly prohibited by law. It is essential to adhere to these guidelines to ensure compliance with legal and ethical standards in research and experimentation.