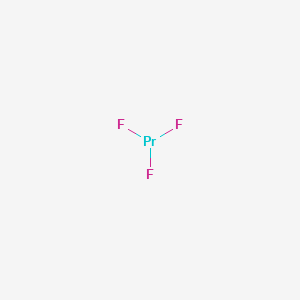
Praseodymium trifluoride
- Click on QUICK INQUIRY to receive a quote from our team of experts.
- With the quality product at a COMPETITIVE price, you can focus more on your research.
Overview
Description
Scientific Research Applications
2.1. Metal Production
Praseodymium trifluoride is used as a precursor in the production of praseodymium metal. The compound serves as a source of praseodymium in various metallurgical processes, particularly in reducing environments where fluorides can facilitate metal extraction .
2.2. Electrochemical Studies
Recent research has explored the use of this compound in molten salt electrolysis processes. Studies indicate that PrF₃ can be employed as an electrolyte for the co-deposition of praseodymium and neodymium metals, enhancing the efficiency of the electrodeposition process at elevated temperatures (around 1050 °C). This method allows for improved metal recovery rates and reduced greenhouse gas emissions during metal production .
2.3. Nuclear Magnetic Resonance Studies
This compound has been utilized in nuclear-quadrupole-resonance studies to investigate the electronic environment of trivalent praseodymium ions within crystalline matrices. Such studies provide insights into the magnetic properties and potential applications of praseodymium compounds in quantum computing and magnetic materials .
3.1. Permanent Magnet Alloys
This compound plays a critical role in the development of rare earth permanent magnet alloys. These alloys are essential for manufacturing high-performance magnets used in electric motors, generators, and various electronic devices .
3.2. Optical Materials
The compound is also applied in producing color glasses and enamels, where it contributes to specific optical properties such as color enhancement and stability under thermal stress. Its unique refractive index makes it suitable for specialized optical applications .
5.1. Electrochemical Co-Deposition Study
A study conducted on the co-deposition of neodymium and praseodymium from a molten fluoride salt bath demonstrated that varying concentrations of PrF₃ significantly influenced deposition rates and metal purity. The results indicated that optimal conditions could lead to higher yields while minimizing environmental impact due to reduced greenhouse gas production during electrolysis .
5.2. Magnetic Properties Investigation
Research into the magnetic properties of praseodymium-based alloys revealed that incorporating this compound enhances the coercivity and remanence of permanent magnets, making them suitable for high-performance applications in electric vehicles and renewable energy systems .
Mechanism of Action
Praseodymium trifluoride can be compared with other praseodymium compounds and rare-earth metal fluorides:
Praseodymium(III) Chloride (PrCl₃): Unlike this compound, praseodymium(III) chloride is a light green solid that is typically prepared by reacting praseodymium metal with hydrogen chloride.
Praseodymium(III) Bromide (PrBr₃): This compound is the only stable bromide of praseodymium and adopts a different crystal structure compared to this compound.
Praseodymium(IV) Fluoride (PrF₄): Praseodymium(IV) fluoride is produced by reacting a mixture of sodium fluoride and praseodymium(III) fluoride with fluorine gas.
Uniqueness:
Comparison with Similar Compounds
Physical and Chemical Properties
Praseodymium trifluoride (PrF₃) is a green, odorless solid that exists in various forms, including powder, flakes, or crystalline structures . It is a lanthanide trifluoride with a YF₃-type crystal structure when reacting with xenon fluorides under specific conditions, enabling the formation of thermally stable 1:4 compounds when praseodymium is oxidized to the +4 state . PrF₃ exhibits slight covalency in its bonding, as evidenced by infrared spectral studies, with minimal involvement of 4f orbitals .
Structural Comparisons
PrF₃’s YF₃-type structure contrasts with the LaF₃ structure observed in lighter lanthanides (e.g., LaF₃).
Table 1: Structural and Reactivity Features
Compound | Crystal Structure | Notable Reactivity | Thermal Stability of XeF₅ Salts |
---|---|---|---|
PrF₃ | YF₃-type | Forms 1:4 XeF₅ salts (Pr⁴⁺) | Stable at RT (1:4 compound) |
LaF₃ | LaF₃-type | No Xe(VI) compounds formed | N/A |
NdF₃ | YF₃-type | Forms 1:2 XeF₅ salts (Nd³⁺) | Stable at RT (1:2 compound) |
Solubility in Aqueous and Molten Salt Systems
PrF₃ exhibits intermediate solubility in aqueous solutions compared to other lanthanide trifluorides. Its solubility product (Ksp) is lower than LaF₃ but higher than LuF₃, reflecting trends in ionic radius and lattice energy across the lanthanide series . In molten fluoride eutectics, PrF₃ demonstrates measurable solubility at high temperatures (e.g., 500–750°C), critical for applications in nuclear reactor coolants or electrochemical synthesis .
Table 2: Solubility Trends in Aqueous Solutions
Compound | Solubility Product (Ksp) | Trend in Lanthanide Series |
---|---|---|
LaF₃ | Higher | Decreases with atomic number |
PrF₃ | Intermediate | |
LuF₃ | Lower |
Thermodynamic Properties
PrF₃ has a heat of sublimation of 103.3–106.6 kcal/mol at 298.15 K, measured via torsion-effusion methods . This value aligns with typical lanthanide trifluorides but is slightly lower than CeF₃ (110 kcal/mol), reflecting differences in bond strength and lattice stability.
Chemical Reactivity and Redox Behavior
Praseodymium’s ability to stabilize the +4 oxidation state in solid-state compounds (e.g., PrF₄) is unique among lighter lanthanides. This redox flexibility enables PrF₃ to participate in reactions forming xenon fluoride salts, unlike LaF₃ or NdF₃, which predominantly retain the +3 state .
Optical and Catalytic Properties
PrF₃-doped crystals exhibit redshifted fluorescence spectra compared to Pr³⁺ in oxygen-coordinated environments, attributed to increased covalent character in Pr–F bonds (0.7% covalency) . In catalysis, nanostructured praseodymium oxides (e.g., Pr₆O₁₁) outperform PrF₃ in ethanol sensing and CO oxidation, highlighting the impact of anion choice on catalytic activity .
Biological Activity
Praseodymium trifluoride (PrF₃) is an inorganic compound that has garnered interest in various scientific fields due to its unique properties and potential applications. This article explores the biological activity of PrF₃, focusing on its interactions with biological systems, potential health effects, and implications for medical and environmental research.
This compound is characterized by its pale green crystalline structure and is known for its stability as a fluoride of praseodymium. Its chemical formula is PrF₃, and it is typically synthesized through reactions involving praseodymium(III) nitrate and sodium fluoride. The compound is insoluble in water, which influences its biological interactions and potential toxicity.
Toxicological Studies
Research into the toxicological effects of rare earth elements, including praseodymium, indicates that exposure to these compounds can lead to various health risks. A study reported elevated levels of praseodymium in individuals living near rare earth mining operations, highlighting concerns about chronic exposure leading to bioaccumulation in human tissues such as blood, urine, and hair .
Sample Type | Praseodymium Levels (μg/L) |
---|---|
Blood | 0.132 |
Urine | 0.055 |
Hair | 0.012 |
These findings suggest that while praseodymium may not be acutely toxic at low levels, long-term exposure could pose risks due to its accumulation.
Histochemical Applications
Praseodymium ions have been utilized in histochemistry as capture agents for phosphatase activity detection. Studies have shown that praseodymium can effectively trap phosphate ions, allowing for the visualization of alkaline phosphatase activity in tissues such as the intestine and kidney . This application underscores the potential use of PrF₃ in biological research and diagnostics.
Fluorescence Spectroscopy
A notable case study involved the use of praseodymium-doped materials in fluorescence spectroscopy. The absorption and fluorescence spectra of praseodymium-doped lanthanum trifluoride revealed significant shifts in energy levels based on the chemical environment surrounding the praseodymium ions . This study demonstrated how PrF₃ could be used to enhance optical properties in biological imaging applications.
Electrochemical Behavior
Another investigation focused on the electrochemical behavior of praseodymium ions in molten salt systems. The study explored the reduction processes of Pr(III) ions, indicating that PrF₃ could be part of efficient electrodeposition techniques for rare earth metals . Understanding these processes is crucial for developing methods to mitigate environmental impacts from rare earth mining.
Research Findings
Absorption Characteristics : The absorption spectra of PrF₃ indicate that it exhibits distinct electronic transitions that can be leveraged for various optical applications. Research has shown that praseodymium ions can enhance luminescence properties when incorporated into glass matrices, making them suitable for temperature sensing applications .
Health Risk Assessments : Ongoing assessments are necessary to evaluate the long-term health effects associated with exposure to praseodymium compounds. Studies suggest a correlation between elevated exposure levels and adverse health outcomes, necessitating further investigation into safe handling practices and regulatory measures .
Q & A
Basic Research Questions
Q. What are the established synthesis routes for praseodymium trifluoride, and how do reaction conditions influence product purity?
this compound can be synthesized via two primary methods:
- Chlorine trifluoride (ClF₃) reaction : Requires controlled gas-phase reactions at elevated temperatures to avoid intermediate byproducts.
- Hydrofluoric acid (HF) precipitation : Involves aqueous-phase reactions with praseodymium salts, demanding strict pH and temperature control to prevent oxide/hydroxide contamination . Purity is validated using X-ray diffraction (XRD) and elemental analysis to confirm phase composition and stoichiometry.
Q. What characterization techniques are essential for verifying the structural and chemical integrity of this compound?
- XRD : Confirms crystalline phase and lattice parameters.
- Thermogravimetric analysis (TGA) : Assesses thermal stability and decomposition thresholds.
- Spectroscopy (UV-Vis, FTIR) : Identifies electronic transitions and ligand bonding environments, particularly for detecting trace oxides or hydroxides .
Q. How is the enthalpy of formation of this compound experimentally determined, and why is this critical for thermodynamic modeling?
Fluorine bomb calorimetry is the gold standard, involving combustion of praseodymium metal in F₂ gas to measure heat release. This data is vital for predicting PrF₃ stability in high-temperature applications like molten salt reactors .
Advanced Research Questions
Q. How does the solubility of this compound in molten fluoride eutectics vary with temperature, and what methods ensure accurate measurement?
In 46.5LiF-11.5NaF-42KF eutectic systems, solubility increases from 500°C to 750°C. Two methodologies are employed:
- Isothermal saturation : Measures equilibrium solubility via gravimetric analysis.
- Reflectance spectroscopy : Provides real-time monitoring of ionic species (e.g., Pr³⁺) in melts, enabling dynamic solubility profiling . Discrepancies between methods highlight the need for cross-validation using XRD or inductively coupled plasma (ICP) analysis.
Q. What spectroscopic techniques resolve contradictions in reported covalent bonding characteristics of Pr³⁺ in trifluoride matrices?
Absorption and fluorescence spectroscopy in matrices like LaF₃ or YAG reveal shifts in J-level centers due to ligand electronegativity. For example, Pr–F bonds exhibit 0.7% lower covalency than Pr–O bonds, impacting optical properties like fluorescence lifetimes . Time-resolved laser spectroscopy further distinguishes ligand-field effects from lattice phonon interactions.
Q. How can phase transformation inconsistencies in this compound under thermal stress be systematically addressed?
Contradictions arise from rapid cooling rates or uncontrolled atmospheres. A combined approach using:
- Controlled thermal treatments : Slow cooling (1–5°C/min) under inert gas to stabilize intermediate phases.
- Synchrotron XRD : Captures real-time structural changes during heating/cooling cycles.
- Oxygen content analysis : WD-XRF or elemental analyzers validate stoichiometric shifts during phase transitions .
Q. What methodologies optimize this compound separation in salt-metal extraction systems for nuclear waste processing?
Distribution coefficients (e.g., PrF₃ in MgCl₂-NaCl-KCl melts) depend on alloy composition. Radiochemical tracer studies with Cu-Mg alloys show 10⁵× higher partitioning efficiency than Al-Mg systems due to praseodymium activity differences. Methodological rigor requires inert-atmosphere gloveboxes and gamma spectroscopy for tracer quantification .
Q. How do ligand environments (e.g., F⁻ vs. O²⁻) influence the catalytic activity of this compound in oxidative reactions?
Comparative studies using X-ray photoelectron spectroscopy (XPS) and density functional theory (DFT) reveal that F⁻ ligands stabilize Pr³⁺ oxidation states, reducing redox activity. In contrast, oxygen-rich environments promote Pr³⁺→Pr⁴⁺ transitions, enhancing catalytic efficiency in methane oxidation .
Q. Data-Driven Research Design Considerations
Q. What experimental parameters are critical for replicating this compound solubility studies in molten salts?
- Temperature uniformity : ±2°C tolerance using resistive furnaces.
- Ion activity monitoring : In-situ electrochemical sensors or spectroscopy.
- Post-experiment quenching : Rapid cooling to "freeze" melt composition for ex-situ analysis .
Q. How should researchers design studies to address gaps in higher fluoride (PrF₄) stability data?
- Fluorination kinetics : React PrF₃ with F₂ gas at 400–600°C, monitored via mass spectrometry.
- High-pressure XRD : Resolves structural transitions during fluorination. Existing discrepancies in PrF₄ stability underscore the need for O₂-free environments to avoid oxyfluoride formation .
Properties
CAS No. |
13709-46-1 |
---|---|
Molecular Formula |
F3Pr |
Molecular Weight |
197.90287 g/mol |
IUPAC Name |
praseodymium(3+);trifluoride |
InChI |
InChI=1S/3FH.Pr/h3*1H;/q;;;+3/p-3 |
InChI Key |
BOTHRHRVFIZTGG-UHFFFAOYSA-K |
SMILES |
F[Pr](F)F |
Canonical SMILES |
[F-].[F-].[F-].[Pr+3] |
Key on ui other cas no. |
13709-46-1 |
Pictograms |
Acute Toxic; Irritant |
Origin of Product |
United States |
Disclaimer and Information on In-Vitro Research Products
Please be aware that all articles and product information presented on BenchChem are intended solely for informational purposes. The products available for purchase on BenchChem are specifically designed for in-vitro studies, which are conducted outside of living organisms. In-vitro studies, derived from the Latin term "in glass," involve experiments performed in controlled laboratory settings using cells or tissues. It is important to note that these products are not categorized as medicines or drugs, and they have not received approval from the FDA for the prevention, treatment, or cure of any medical condition, ailment, or disease. We must emphasize that any form of bodily introduction of these products into humans or animals is strictly prohibited by law. It is essential to adhere to these guidelines to ensure compliance with legal and ethical standards in research and experimentation.