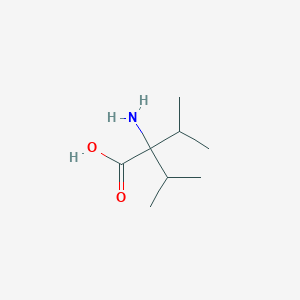
alpha,alpha-Diisopropylglycine
- Click on QUICK INQUIRY to receive a quote from our team of experts.
- With the quality product at a COMPETITIVE price, you can focus more on your research.
Overview
Description
It contains an α-amino group, an α-carboxylic acid group, and a side chain isopropyl group, making it a non-polar aliphatic amino acid . Valine is essential in humans, meaning the body cannot synthesize it; it must be obtained from dietary sources such as meats, dairy products, soy products, beans, and legumes .
Preparation Methods
Synthetic Routes and Reaction Conditions
Valine can be synthesized through several steps starting from pyruvic acid. The initial part of the pathway also leads to leucine. The intermediate α-ketoisovalerate undergoes reductive amination with glutamate . Industrial production methods often involve fermentation processes using bacteria or yeast that have been genetically modified to overproduce valine .
Chemical Reactions Analysis
Types of Reactions
Valine undergoes various chemical reactions, including:
Oxidation: Valine can be oxidized to form α-ketoisovalerate.
Reduction: Reduction of valine can lead to the formation of isobutyraldehyde.
Substitution: Valine can participate in substitution reactions where the amino group is replaced by other functional groups.
Common Reagents and Conditions
Oxidation: Common oxidizing agents include potassium permanganate (KMnO₄) and hydrogen peroxide (H₂O₂).
Reduction: Reducing agents such as sodium borohydride (NaBH₄) or lithium aluminum hydride (LiAlH₄) are used.
Substitution: Reagents like halogens (e.g., chlorine, bromine) and nucleophiles (e.g., ammonia, amines) are commonly used.
Major Products
Oxidation: α-Ketoisovalerate
Reduction: Isobutyraldehyde
Substitution: Various substituted valine derivatives depending on the reagents used.
Scientific Research Applications
Valine has numerous applications in scientific research:
Chemistry: Used as a building block in peptide synthesis and as a chiral auxiliary in asymmetric synthesis.
Biology: Plays a crucial role in protein synthesis and is involved in various metabolic pathways.
Industry: Used in the production of dietary supplements and as a feed additive in animal nutrition.
Mechanism of Action
Valine exerts its effects primarily through its role in protein synthesis. It is incorporated into proteins during translation, where it interacts with ribosomes and transfer RNA (tRNA). Valine also plays a role in the regulation of blood sugar levels and the stimulation of muscle growth and repair . The molecular targets and pathways involved include the mTOR pathway, which is crucial for cell growth and metabolism .
Comparison with Similar Compounds
Valine is similar to other branched-chain amino acids (BCAAs) such as leucine and isoleucine. valine is unique in its specific role in muscle metabolism and its ability to be converted into glucose via gluconeogenesis . Similar compounds include:
Leucine: Another BCAA involved in protein synthesis and muscle repair.
Isoleucine: A BCAA that plays a role in energy production and immune function.
Valine’s uniqueness lies in its specific metabolic pathways and its essential role in human nutrition .
Properties
Molecular Formula |
C8H17NO2 |
---|---|
Molecular Weight |
159.23 g/mol |
IUPAC Name |
2-amino-3-methyl-2-propan-2-ylbutanoic acid |
InChI |
InChI=1S/C8H17NO2/c1-5(2)8(9,6(3)4)7(10)11/h5-6H,9H2,1-4H3,(H,10,11) |
InChI Key |
OUBVKSUTYWBLCS-UHFFFAOYSA-N |
Canonical SMILES |
CC(C)C(C(C)C)(C(=O)O)N |
Origin of Product |
United States |
Disclaimer and Information on In-Vitro Research Products
Please be aware that all articles and product information presented on BenchChem are intended solely for informational purposes. The products available for purchase on BenchChem are specifically designed for in-vitro studies, which are conducted outside of living organisms. In-vitro studies, derived from the Latin term "in glass," involve experiments performed in controlled laboratory settings using cells or tissues. It is important to note that these products are not categorized as medicines or drugs, and they have not received approval from the FDA for the prevention, treatment, or cure of any medical condition, ailment, or disease. We must emphasize that any form of bodily introduction of these products into humans or animals is strictly prohibited by law. It is essential to adhere to these guidelines to ensure compliance with legal and ethical standards in research and experimentation.