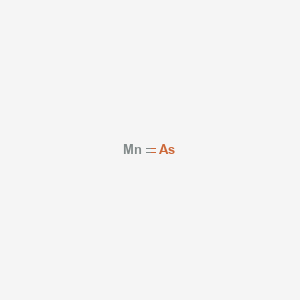
Manganese arsenide
Overview
Description
Manganese arsenide (MnAs) is an intermetallic compound with a hexagonal crystal structure, notable for its first-order phase transition near room temperature and ferromagnetic properties in thin-film forms . It exists in multiple stoichiometric forms, including MnAs (CAS 12005-96-8), Mn₂As, and Mn₃As₂, each exhibiting distinct structural and magnetic behaviors . Bulk MnAs has been historically studied for its magnetic transitions, while epitaxial MnAs thin films on semiconductors like GaAs have gained attention in spintronics due to their compatibility with existing semiconductor technologies .
Preparation Methods
Synthetic Routes and Reaction Conditions: Manganese arsenide can be synthesized through various methods, including solid-state reactions and chemical vapor deposition. One common method involves the direct reaction of manganese and arsenic at high temperatures. The reaction is typically carried out in a sealed tube to prevent the loss of arsenic due to its high vapor pressure .
Industrial Production Methods: Industrial production of this compound often involves the reduction of manganese oxide with arsenic trioxide in the presence of a reducing agent such as hydrogen. This method ensures the production of high-purity this compound suitable for industrial applications .
Chemical Reactions Analysis
Types of Reactions: Manganese arsenide undergoes various chemical reactions, including oxidation, reduction, and substitution reactions.
Common Reagents and Conditions:
Major Products: The major products formed from these reactions include various oxides of manganese and arsenic, as well as new intermetallic compounds depending on the specific reaction conditions .
Scientific Research Applications
Semiconductors
MnAs is primarily used in semiconductor applications due to its electronic properties. It can be integrated into devices such as:
- Transistors : MnAs can serve as a channel material in field-effect transistors (FETs), leveraging its semiconductor properties.
- Laser Diodes : The compound's ability to emit light when electrically stimulated makes it suitable for laser diode applications .
Spintronics
Spintronics, or spin electronics, exploits the intrinsic spin of electrons alongside their charge to develop devices with enhanced functionality. MnAs plays a pivotal role in this field:
- Magnetic Sensors : Its ferromagnetic properties enable the development of sensitive magnetic sensors for various applications, including data storage and retrieval systems .
- Data Storage Devices : MnAs is being explored for use in next-generation data storage technologies due to its ability to manipulate electron spins effectively .
Magnetic Refrigeration
The unique magnetic properties of MnAs allow it to be used in magnetic refrigeration systems. This technology utilizes the magnetocaloric effect, where materials exhibit temperature changes under magnetic fields, providing an energy-efficient cooling method .
Photonic Applications
MnAs has potential applications in photonic devices due to its optical properties:
- Quantum Dots : The compound can be used to create quantum dots, which are essential for advanced photonic applications like light-emitting diodes (LEDs) and solar cells .
- Optical Sensors : Its sensitivity to light makes MnAs suitable for developing optical sensors that can detect changes in environmental conditions .
Case Study 1: Transducer Applications
Research conducted at General Electric highlighted the use of MnAs as a transducer material. The study demonstrated that MnAs undergoes significant volume changes when subjected to strong magnetic fields, which can be harnessed in sonar projector designs. The findings indicated that these properties could lead to innovative designs for acoustic transducers used in underwater exploration .
Case Study 2: In Situ Growth Techniques
A study from Lund University investigated the growth of MnAs nanostructures using environmental transmission electron microscopy (ETEM). This research focused on optimizing synthesis parameters to control the growth rate and structural transitions of MnAs. The results showed promise for integrating MnAs with other semiconductor materials, paving the way for advanced nanotechnology applications .
Summary Table of Applications
Application Area | Specific Uses | Key Properties Utilized |
---|---|---|
Semiconductors | Transistors, Laser Diodes | Semiconductor behavior |
Spintronics | Magnetic Sensors, Data Storage Devices | Ferromagnetism, Spin manipulation |
Magnetic Refrigeration | Cooling Systems | Magnetocaloric effect |
Photonic Applications | Quantum Dots, Optical Sensors | Optical sensitivity |
Mechanism of Action
The mechanism by which manganese arsenide exerts its effects is primarily related to its ferromagnetic properties. The compound’s ability to transition between ferromagnetic and paramagnetic phases allows it to be used in various magnetic applications. In spintronics, this compound facilitates the injection of spin-polarized electrons into semiconductors, enhancing the performance of spintronic devices . In cancer therapy, this compound nanoparticles target the acidic tumor microenvironment, releasing arsenic trioxide and manganese ions to induce apoptosis in cancer cells .
Comparison with Similar Compounds
Structural and Magnetic Properties
Key Research Controversies and Advances
- This contrasts with thin-film studies confirming robust ferromagnetism .
- GaMnAS Doping Efficiency : Optimal Mn doping levels (10–20%) maximize carrier mobility (10⁴ cm²/V·s) and Hall resistivity (3.6 mΩ·cm), but higher doping (>20%) introduces defects that degrade performance .
Biological Activity
Manganese arsenide (MnAs) is a compound of significant interest due to its unique properties and potential biological implications. This article explores the biological activity of MnAs, focusing on its mechanisms of toxicity, interactions with biological systems, and implications for health.
This compound is characterized by its antiferromagnetic properties and is primarily studied in the context of its magnetic behavior and potential applications in electronics. Studies have shown that MnAs undergoes a magnetic phase transition at specific temperatures, which influences its physical properties . The compound's structure can be affected by various factors, including preparation methods and environmental conditions, which may also impact its biological interactions.
Mechanisms of Toxicity
The biological activity of this compound can be linked to the toxicological profiles of both manganese and arsenic. Chronic exposure to these metals has been associated with several adverse health effects, primarily through mechanisms such as:
- Oxidative Stress : Both manganese and arsenic can generate reactive oxygen species (ROS), leading to oxidative damage in cells. This oxidative stress can disrupt cellular functions and contribute to neurodegenerative diseases .
- Hematopoietic Interference : Manganese has been shown to interfere with heme synthesis, affecting the production of hemoglobin and potentially leading to anemia. This interference occurs through the inhibition of key enzymes in the heme biosynthetic pathway .
- Neurotoxicity : Exposure to MnAs has been linked to neurotoxic effects, including cognitive deficits and motor dysfunction. The shared pathways of toxicity among lead, arsenic, and manganese suggest a cumulative risk when exposed to metal mixtures .
In Vitro and In Vivo Research
Research has highlighted various aspects of MnAs's biological activity:
- Cellular Studies : In vitro studies have demonstrated that MnAs can induce cytotoxic effects in neuronal cell lines, leading to increased apoptosis rates. These findings suggest that exposure to MnAs may compromise neuronal integrity .
- Animal Models : Animal studies have shown that chronic exposure to manganese compounds can lead to behavioral changes indicative of neurotoxicity. For instance, rats exposed to high levels of manganese exhibited impaired motor coordination and learning deficits .
- Nanoparticle Applications : Recent investigations into MnAs nanoparticles have revealed potential applications in nanomedicine, particularly in cancer therapy. These nanoparticles have demonstrated enhanced radiotherapeutic efficacy by acting as radiosensitizers in tumor environments .
Occupational Exposure
A notable case study involved workers in industries where manganese and arsenic were prevalent. Longitudinal studies indicated that these workers exhibited higher rates of neurological disorders compared to control groups. The findings emphasized the need for monitoring occupational exposure limits and implementing protective measures .
Environmental Impact
Another study focused on communities near mining operations where this compound was released into the environment. Residents showed elevated levels of manganese in their blood, correlating with increased respiratory issues and cognitive impairments. This underscores the environmental health risks associated with MnAs exposure .
Data Summary
The following table summarizes key findings related to the biological activity of this compound:
Study Type | Findings | Implications |
---|---|---|
In Vitro | Induced cytotoxicity in neuronal cells | Potential neurotoxic effects |
Animal Studies | Behavioral changes in rats due to chronic manganese exposure | Risk of neurodegenerative diseases |
Occupational Study | Higher neurological disorder rates among exposed workers | Need for stricter occupational safety regulations |
Environmental Study | Elevated blood manganese levels linked to respiratory issues | Environmental health monitoring required |
Q & A
Q. How can the crystal structure of manganese arsenide be accurately determined using X-ray diffraction (XRD), and what methodological considerations are critical for minimizing errors?
Basic Research Question
To determine the crystal structure, use high-resolution XRD with monochromatic Cu-Kα radiation (λ = 1.5406 Å). Ensure sample homogeneity by grinding to <50 µm particle size and mounting on a zero-background holder. Collect data over a 2θ range of 10–90° with a step size of 0.02°. Employ Rietveld refinement using software like FullProf or TOPAS, incorporating anisotropic displacement parameters and accounting for preferred orientation . Validate results against known ICSD entries (e.g., MnAs, P63/mmc space group) and cross-check with neutron diffraction if magnetic scattering is observed.
Q. What synthesis methodologies are optimal for producing high-purity this compound, and how can stoichiometric deviations be quantified?
Basic Research Question
Solid-state synthesis under inert argon atmosphere (800–1000°C, 48–72 hours) is standard. Use stoichiometric Mn:As ratios (1:1) with elemental powders (99.99% purity). Post-synthesis, perform energy-dispersive X-ray spectroscopy (EDS) to quantify Mn/As ratios. For trace impurity analysis, employ inductively coupled plasma mass spectrometry (ICP-MS). Thermogravimetric analysis (TGA) under argon can identify unreacted As via mass loss at 614°C (sublimation) .
Q. How can contradictions in reported magnetic properties of this compound (e.g., Curie temperature variability) be systematically resolved?
Advanced Research Question
Contradictions often arise from sample purity, measurement techniques, or stoichiometric variations. Conduct comparative studies using:
- SQUID magnetometry : Measure magnetization loops at 2–400 K with field cooling (FC) and zero-field cooling (ZFC) protocols.
- Mössbauer spectroscopy : Probe local magnetic environments of Mn sites.
- Neutron scattering : Resolve magnetic structure and spin interactions.
Cross-reference data with synchrotron X-ray absorption spectroscopy (XAS) to detect oxidation states. Statistical meta-analysis of published datasets (e.g., using PRISMA guidelines) can identify systematic biases .
Q. What experimental protocols optimize scanning tunneling microscopy (STM) for studying charge-switching behavior in this compound?
Advanced Research Question
Use ultra-high vacuum (UHV) STM (<1×10<sup>−10</sup> mbar) with electrochemically etched tungsten tips. Calibrate tip work function via field emission resonance (FER) on Au(111). For MnAs(001) surfaces, acquire dI/dV spectra at 4.2 K to map local density of states (LDOS). Apply bias voltages ±2 V with 10 pA tunneling current. Analyze charge-switching events using Poisson statistics to distinguish intrinsic behavior from tip-induced effects .
Q. How should researchers address inconsistencies in thermal conductivity measurements of this compound across studies?
Advanced Research Question
Inconsistencies may stem from phonon scattering mechanisms or anisotropic thermal transport. Use:
- Time-domain thermoreflectance (TDTR) : Measure in-plane vs. cross-plane conductivity.
- Ab initio calculations : Compute phonon dispersion using density functional theory (DFT) with Perdew-Burke-Ernzerhof (PBE) functionals.
Apply Bayesian regression to quantify uncertainty in experimental parameters (e.g., laser spot size, interface resistance) .
Q. What role does stoichiometry play in the phase stability of this compound, and how can phase transitions be experimentally controlled?
Advanced Research Question
MnAs exhibits a hexagonal (NiAs-type) to orthorhombic (MnP-type) transition at ~45°C. Control stoichiometry via flux growth (e.g., using Sn flux at 600°C). Monitor phase purity via high-temperature XRD (HT-XRD) and differential scanning calorimetry (DSC). For epitaxial films, use molecular beam epitaxy (MBE) with in-situ reflection high-energy electron diffraction (RHEED) to stabilize desired phases .
Q. What methodologies ensure accurate measurement of this compound’s electronic band structure using angle-resolved photoemission spectroscopy (ARPES)?
Advanced Research Question
Use synchrotron-based ARPES with photon energies 20–100 eV. Clean surfaces via sputtering (Ar<sup>+</sup>, 1 keV) and annealing (300°C). Map Fermi surfaces using <1 meV energy resolution. Correct for sample charging with a flood gun. Validate against GW approximation calculations to account for quasiparticle effects .
Q. How can researchers reconcile discrepancies in reported bandgap values of this compound from optical and electrical measurements?
Advanced Research Question
Optical bandgaps (from UV-Vis or ellipsometry) may differ from transport-derived values due to excitonic effects or defect states. Perform:
- Spectroscopic ellipsometry : Fit dielectric function using Tauc-Lorentz models.
- Hall effect measurements : Extract carrier concentration and mobility to calculate effective mass and bandgap via the Kane model.
Compare with DFT+U calculations (U = 3–5 eV for Mn 3d states) .
Q. What statistical approaches are recommended for analyzing heterogeneous datasets in this compound research (e.g., conflicting dopant effects)?
Advanced Research Question
Apply principal component analysis (PCA) to reduce dimensionality of datasets (e.g., magnetic, structural, electronic). Use hierarchical clustering to group studies by synthesis method or measurement technique. For meta-analysis, employ random-effects models to account for between-study variance. Open-source tools like R or Python’s SciPy suite enable reproducible workflows .
Q. How should researchers design experiments to probe the interplay between spin-orbit coupling and magnetism in this compound?
Advanced Research Question
Combine polarized neutron diffraction (to resolve spin textures) with X-ray magnetic circular dichroism (XMCD) at Mn L-edges. Use femtosecond laser pulses to trigger ultrafast demagnetization and measure time-resolved magneto-optical Kerr effect (TR-MOKE). Theoretical modeling via relativistic DFT (including spin-orbit terms) can predict anisotropy energies .
Properties
InChI |
InChI=1S/As.Mn | |
---|---|---|
Source | PubChem | |
URL | https://pubchem.ncbi.nlm.nih.gov | |
Description | Data deposited in or computed by PubChem | |
InChI Key |
NYOGMBUMDPBEJK-UHFFFAOYSA-N | |
Source | PubChem | |
URL | https://pubchem.ncbi.nlm.nih.gov | |
Description | Data deposited in or computed by PubChem | |
Canonical SMILES |
[Mn]=[As] | |
Source | PubChem | |
URL | https://pubchem.ncbi.nlm.nih.gov | |
Description | Data deposited in or computed by PubChem | |
Molecular Formula |
AsMn | |
Source | PubChem | |
URL | https://pubchem.ncbi.nlm.nih.gov | |
Description | Data deposited in or computed by PubChem | |
DSSTOX Substance ID |
DTXSID001312125 | |
Record name | Manganese arsenide (MnAs) | |
Source | EPA DSSTox | |
URL | https://comptox.epa.gov/dashboard/DTXSID001312125 | |
Description | DSSTox provides a high quality public chemistry resource for supporting improved predictive toxicology. | |
Molecular Weight |
129.85964 g/mol | |
Source | PubChem | |
URL | https://pubchem.ncbi.nlm.nih.gov | |
Description | Data deposited in or computed by PubChem | |
CAS No. |
12005-96-8, 12005-95-7, 61219-26-9 | |
Record name | Manganese arsenide (Mn2As) | |
Source | CAS Common Chemistry | |
URL | https://commonchemistry.cas.org/detail?cas_rn=12005-96-8 | |
Description | CAS Common Chemistry is an open community resource for accessing chemical information. Nearly 500,000 chemical substances from CAS REGISTRY cover areas of community interest, including common and frequently regulated chemicals, and those relevant to high school and undergraduate chemistry classes. This chemical information, curated by our expert scientists, is provided in alignment with our mission as a division of the American Chemical Society. | |
Explanation | The data from CAS Common Chemistry is provided under a CC-BY-NC 4.0 license, unless otherwise stated. | |
Record name | Manganese arsenide (MnAs) | |
Source | CAS Common Chemistry | |
URL | https://commonchemistry.cas.org/detail?cas_rn=12005-95-7 | |
Description | CAS Common Chemistry is an open community resource for accessing chemical information. Nearly 500,000 chemical substances from CAS REGISTRY cover areas of community interest, including common and frequently regulated chemicals, and those relevant to high school and undergraduate chemistry classes. This chemical information, curated by our expert scientists, is provided in alignment with our mission as a division of the American Chemical Society. | |
Explanation | The data from CAS Common Chemistry is provided under a CC-BY-NC 4.0 license, unless otherwise stated. | |
Record name | Manganese arsenide (Mn3As) | |
Source | CAS Common Chemistry | |
URL | https://commonchemistry.cas.org/detail?cas_rn=61219-26-9 | |
Description | CAS Common Chemistry is an open community resource for accessing chemical information. Nearly 500,000 chemical substances from CAS REGISTRY cover areas of community interest, including common and frequently regulated chemicals, and those relevant to high school and undergraduate chemistry classes. This chemical information, curated by our expert scientists, is provided in alignment with our mission as a division of the American Chemical Society. | |
Explanation | The data from CAS Common Chemistry is provided under a CC-BY-NC 4.0 license, unless otherwise stated. | |
Record name | Manganese arsenide (MnAs) | |
Source | ChemIDplus | |
URL | https://pubchem.ncbi.nlm.nih.gov/substance/?source=chemidplus&sourceid=0012005957 | |
Description | ChemIDplus is a free, web search system that provides access to the structure and nomenclature authority files used for the identification of chemical substances cited in National Library of Medicine (NLM) databases, including the TOXNET system. | |
Record name | Manganese arsenide (MnAs) | |
Source | EPA Chemicals under the TSCA | |
URL | https://www.epa.gov/chemicals-under-tsca | |
Description | EPA Chemicals under the Toxic Substances Control Act (TSCA) collection contains information on chemicals and their regulations under TSCA, including non-confidential content from the TSCA Chemical Substance Inventory and Chemical Data Reporting. | |
Record name | Manganese arsenide (MnAs) | |
Source | EPA DSSTox | |
URL | https://comptox.epa.gov/dashboard/DTXSID001312125 | |
Description | DSSTox provides a high quality public chemistry resource for supporting improved predictive toxicology. | |
Record name | Manganese arsenide | |
Source | European Chemicals Agency (ECHA) | |
URL | https://echa.europa.eu/substance-information/-/substanceinfo/100.031.331 | |
Description | The European Chemicals Agency (ECHA) is an agency of the European Union which is the driving force among regulatory authorities in implementing the EU's groundbreaking chemicals legislation for the benefit of human health and the environment as well as for innovation and competitiveness. | |
Explanation | Use of the information, documents and data from the ECHA website is subject to the terms and conditions of this Legal Notice, and subject to other binding limitations provided for under applicable law, the information, documents and data made available on the ECHA website may be reproduced, distributed and/or used, totally or in part, for non-commercial purposes provided that ECHA is acknowledged as the source: "Source: European Chemicals Agency, http://echa.europa.eu/". Such acknowledgement must be included in each copy of the material. ECHA permits and encourages organisations and individuals to create links to the ECHA website under the following cumulative conditions: Links can only be made to webpages that provide a link to the Legal Notice page. | |
Disclaimer and Information on In-Vitro Research Products
Please be aware that all articles and product information presented on BenchChem are intended solely for informational purposes. The products available for purchase on BenchChem are specifically designed for in-vitro studies, which are conducted outside of living organisms. In-vitro studies, derived from the Latin term "in glass," involve experiments performed in controlled laboratory settings using cells or tissues. It is important to note that these products are not categorized as medicines or drugs, and they have not received approval from the FDA for the prevention, treatment, or cure of any medical condition, ailment, or disease. We must emphasize that any form of bodily introduction of these products into humans or animals is strictly prohibited by law. It is essential to adhere to these guidelines to ensure compliance with legal and ethical standards in research and experimentation.