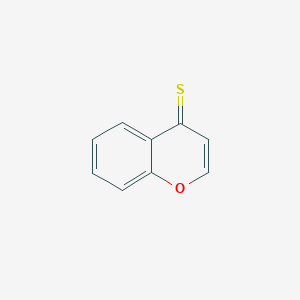
Thiochromone
- Click on QUICK INQUIRY to receive a quote from our team of experts.
- With the quality product at a COMPETITIVE price, you can focus more on your research.
Overview
Description
Preparation Methods
Synthetic Routes and Reaction Conditions: Thiochromone can be synthesized through various methods. One common approach involves the one-pot three-component condensation reaction of aldehydes, malononitrile, and 1,3-diketones in the presence of a basic catalyst such as triethanolamine. This method is advantageous due to its rapid reaction times, high yields, and eco-friendly conditions .
Industrial Production Methods: While specific industrial production methods for this compound are not extensively documented, the principles of green chemistry and efficient catalysis are likely employed to optimize yield and minimize environmental impact.
Chemical Reactions Analysis
Conjugate Addition Reactions
Thiochromone undergoes 1,4-conjugate additions with Grignard reagents under copper catalysis. Key findings include:
-
Catalytic System : CuCN·2LiCl (0.2 equiv) with TMSCl as an activator in THF at 0°C to room temperature .
-
Substrate Scope :
Grignard Reagent Yield (%) Notes n-BuMgCl 89 Optimal conditions i-PrMgCl 69 Steric hindrance reduces yield PhMgBr 85 Aromatic Grignard compatibility 2-FurylMgBr 79 Heterocyclic compatibility -
Mechanistic Insight : The reaction proceeds via copper-mediated activation of the this compound carbonyl, enabling nucleophilic attack by the Grignard reagent at the β-position .
Cyclization and Annulation Reactions
This compound derivatives are synthesized through cyclization strategies:
-
Friedel-Crafts Acylation : Alkynes react with benzoyl chlorides in a one-pot method to yield thiochromenones (e.g., 65–78% yields) .
-
Rhodium-Catalyzed Carbonylative Annulation : Terminal alkynes and aromatic sulfides form thiochromenones via [3+2+1] annulation (13612–13617 examples) .
-
Copper-Catalyzed Ring-Opening : Donor-acceptor cyclopropanes with xanthates yield 3-alkyl-carbonated thioflavones (6648–6652 cases) .
Cross-Coupling Reactions
Palladium-catalyzed cross-couplings enable functionalization:
-
Suzuki-Miyaura Coupling : 2-Sulfinyl-thiochromones react with arylboronic acids (Pd(OAc)₂/XPhos) to form 2-aryl-4H-thiochromen-4-ones (41–66% yields) .
Substituent on this compound Yield (%) Electron-donating (e.g., -OMe) 58–66 Electron-withdrawing (e.g., -NO₂) 41–54 -
Functional Group Tolerance : Halogens (-F, -Cl, -Br) and heteroaryl groups (e.g., pyridyl) are compatible .
Ring-Opening and Functionalization
This compound derivatives undergo further transformations:
-
Reduction : Sodium borohydride reduces thioflavanones to alcohols (81–85% yields) .
-
Oxidation : m-CPBA oxidizes thiochromones to sulfones (70–74% yields) .
-
Halogenation : NCS chlorinates thiochromones at the α-position (65–70% yields) .
This compound’s versatility in conjugate additions, cyclizations, and cross-couplings makes it invaluable for synthesizing sulfur heterocycles with applications in medicinal chemistry. Catalytic systems (Cu, Rh, Pd) and optimized conditions (solvents, additives) are critical for achieving high yields and selectivity.
Scientific Research Applications
Medicinal Chemistry Applications
Thiochromones are recognized for their potential as pharmacologically active compounds. They serve as scaffolds for synthesizing various bioactive molecules, particularly in the development of drugs targeting neurodegenerative diseases and infectious diseases.
Inhibition of Monoamine Oxidase B
Recent studies have highlighted the efficacy of C-3 isoxazole substituted thiochromone S,S-dioxide derivatives as potent inhibitors of human Monoamine Oxidase B (hMAO-B). These compounds exhibit single-digit nanomolar potency and favorable cytotoxicity profiles, making them promising candidates for treating conditions like Parkinson's disease .
Antileishmanial Activity
A series of this compound derivatives have been synthesized and evaluated for their antileishmanial activity against Leishmania panamensis. Compounds with a vinyl sulfone moiety demonstrated high potency (EC50 values < 10 μM) and selectivity indices over 100, indicating their potential as therapeutic agents against leishmaniasis .
Anticancer Properties
Thiochromones are also being explored for their anticancer properties. Their structural similarity to chromones allows them to act as bioisosteres, potentially enhancing the bioactivity of existing chromone-based drugs . Studies have shown that certain this compound derivatives exhibit significant cytotoxic effects on cancer cell lines.
Biological Applications
Thiochromones possess unique photophysical properties that make them suitable for various biological applications.
Photophysical Studies
The ability of thiochromones to participate in photophysical processes enables their use in studying molecular interactions and dynamics. Their stable complexes with water molecules lead to significant changes in electronic states, facilitating research in photochemistry and molecular biology.
Interaction with Parasites
Recent research indicates that thiochromones can disrupt metabolic pathways in parasites responsible for tropical diseases such as malaria and trypanosomiasis. The core structure of 4H-thiochromen-4-one 1,1-dioxide has been identified as an allosteric modulator, interacting with key amino acids in parasite proteins .
Material Science Applications
Thiochromones are not limited to biological applications; they also play a role in materials science.
Organic Light-Emitting Diodes (OLEDs)
Due to their characteristic photo-physical behavior, thiochromones are being investigated for use in organic light-emitting diodes (OLEDs). Their efficient biomolecular photoreaction properties make them suitable candidates for developing advanced optoelectronic materials .
Laser Dyes and Fluorescent Probes
Thiochromones have been employed in developing laser dyes and fluorescent probes due to their stability under light exposure and efficient light emission properties. This application is particularly valuable in biochemical assays and imaging techniques .
Synthesis and Mechanisms
The synthesis of thiochromones involves various methods, including:
- Michael Addition : A common synthetic route where thiochromones are formed through the addition of nucleophiles to α,β-unsaturated carbonyl compounds.
- Cycloaddition Reactions : Diels–Alder reactions have been utilized to create complex thiochromene structures from simpler precursors .
The mechanisms underlying these reactions often involve the formation of intermediates that facilitate the construction of the this compound framework.
Mechanism of Action
The mechanism of action of Thiochromone involves its ability to form hydrogen bonds and participate in various photophysical processes. The compound can form stable complexes with water molecules, leading to significant changes in its electronic states. Upon excitation, the compound undergoes efficient internal conversion and intersystem crossing, which are key processes in its photophysical behavior .
Comparison with Similar Compounds
4H-1-Benzopyran-4-one:
4H-1-Benzothiopyran-4-one: This compound has a sulfur atom in the pyran ring, similar to Thiochromone, but differs in its overall structure and properties.
Uniqueness: this compound is unique due to its thione group, which imparts distinct chemical reactivity and photophysical properties. This makes it particularly valuable in applications requiring specific electronic and bonding characteristics.
Q & A
Basic Research Questions
Q. What are the key synthetic methodologies for thiochromone derivatives, and how can reaction conditions be optimized?
this compound synthesis often involves conjugate addition reactions, cyclization, or functionalization of precursor molecules. For example, lithium dialkylcuprates require Lewis acid additives (e.g., TMSCl) to facilitate conjugate addition to this compound, as unmodified reagents yield negligible products . Optimization strategies include:
- Additive Screening : TMSI and TMSOTf improve yields (up to 85%) by activating the this compound carbonyl group .
- Reagent Reactivity : Gilman reagents (e.g., n-Bu₂CuLi) show limited efficacy compared to cyanocuprates, necessitating tailored reagent selection .
- Spectroscopic Validation : Use ¹H NMR to distinguish thiocoumarins (δH < 8.0 ppm) from thiochromones (δH ~8.5 ppm) via deshielding effects from the carbonyl group .
Q. How can spectroscopic techniques characterize this compound derivatives, and what are critical spectral markers?
Key spectral features include:
- IR Spectroscopy : Thiochromones exhibit a carbonyl stretch at 1610–1630 cm⁻¹, distinct from chromones (1650–1670 cm⁻¹) due to sulfur lone-pair delocalization .
- ¹H NMR : H(5) in thiochromones resonates near δH 8.5 ppm, a diagnostic peak absent in thiocoumarins .
- X-ray Crystallography : Confirms structural features like S1–C2 bond length (1.712 Å) and resonance stabilization in formylthis compound derivatives .
Q. What preliminary biological screening approaches are used to evaluate this compound bioactivity?
- In Vitro Assays : Antimalarial activity against Plasmodium falciparum is assessed via IC₅₀ measurements .
- Enzyme Inhibition : Screen derivatives against targets like Leishmania major N-myristoyltransferase (Lm-NMT) using molecular docking and in-silico analysis .
- Antifungal Testing : Evaluate 2-triazolyl this compound derivatives for inhibitory effects on fungal growth via MIC assays .
Advanced Research Questions
Q. How can computational tools like CADD enhance the design of this compound-based therapeutics?
- Molecular Docking : CADD identifies this compound analogs with high binding affinity to targets like Lm-NMT. For example, amino-acid conjugates show improved hydrophobic interactions in silico .
- QSAR Modeling : Correlate structural features (e.g., electron-withdrawing substituents) with antimalarial activity to guide synthetic prioritization .
- ADMET Prediction : Use tools like SwissADME to optimize pharmacokinetic properties, reducing late-stage attrition .
Q. How can researchers resolve contradictions in this compound bioactivity data across studies?
- Mechanistic Profiling : Compare inhibition kinetics (e.g., Kᵢ values) for enzymes like NMT to distinguish target-specific vs. off-target effects .
- Redox Activity Screening : Assess whether antioxidant/pro-oxidant properties (common in sulfur-containing compounds) confound cellular assays .
- Strain-Specific Testing : Evaluate bioactivity against multiple Plasmodium or fungal strains to rule out resistance-related discrepancies .
Q. What strategies improve synthetic yields of this compound derivatives in challenging reactions?
- Lewis Acid Optimization : Replace TMSCl with TMSOTf to enhance electrophilicity of the carbonyl group in conjugate additions .
- Microwave-Assisted Synthesis : Reduce reaction times and improve regioselectivity in cyclization steps .
- Protecting Group Chemistry : Use photolabile groups (e.g., this compound S,S-dioxides) to temporarily mask reactive sites during functionalization .
Q. How can thiochromones be leveraged in non-therapeutic applications, such as photolabile protecting groups?
- Photoreactivity Tuning : Modify 3-aryl substituents to adjust UV absorption profiles, enabling controlled release of protected molecules (e.g., neurotransmitters) under specific wavelengths .
- Stability Testing : Assess hydrolytic and thermal stability of this compound S,S-dioxides in buffer systems to validate utility in bioorthogonal chemistry .
Q. Methodological Considerations
Q. What frameworks ensure rigorous formulation of this compound-related research questions?
- FINER Criteria : Ensure questions are Feasible, Interesting, Novel, Ethical, and Relevant. For example:
- Novelty: "Does this compound’s sulfur-mediated redox activity enhance its antimalarial mechanism compared to chromones?" .
- PICO Framework : Define Population (e.g., enzyme targets), Intervention (this compound derivatives), Comparison (existing inhibitors), and Outcomes (IC₅₀, selectivity) .
Q. How should researchers manage contradictory data in spectroscopic or biological studies?
Properties
Molecular Formula |
C9H6OS |
---|---|
Molecular Weight |
162.21 g/mol |
IUPAC Name |
chromene-4-thione |
InChI |
InChI=1S/C9H6OS/c11-9-5-6-10-8-4-2-1-3-7(8)9/h1-6H |
InChI Key |
FLGZHHJNRZUPIL-UHFFFAOYSA-N |
Canonical SMILES |
C1=CC=C2C(=C1)C(=S)C=CO2 |
Origin of Product |
United States |
Disclaimer and Information on In-Vitro Research Products
Please be aware that all articles and product information presented on BenchChem are intended solely for informational purposes. The products available for purchase on BenchChem are specifically designed for in-vitro studies, which are conducted outside of living organisms. In-vitro studies, derived from the Latin term "in glass," involve experiments performed in controlled laboratory settings using cells or tissues. It is important to note that these products are not categorized as medicines or drugs, and they have not received approval from the FDA for the prevention, treatment, or cure of any medical condition, ailment, or disease. We must emphasize that any form of bodily introduction of these products into humans or animals is strictly prohibited by law. It is essential to adhere to these guidelines to ensure compliance with legal and ethical standards in research and experimentation.