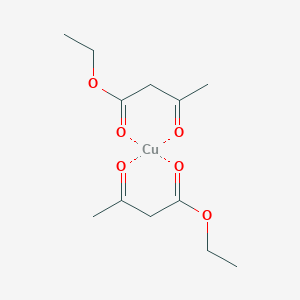
Copper;ethyl 3-oxobutanoate
- Click on QUICK INQUIRY to receive a quote from our team of experts.
- With the quality product at a COMPETITIVE price, you can focus more on your research.
Overview
Description
Ethyl 3-oxobutanoate (IUPAC name), commonly known as ethyl acetoacetate, is a β-keto ester with the molecular formula C₆H₁₀O₃. It is a colorless liquid with a fruity odor and serves as a pivotal intermediate in organic synthesis due to its ability to form enolates, enabling diverse reactions such as Claisen condensations, alkylations, and Michael additions . The ester is more stable than its acid counterpart (3-oxobutanoic acid), making it indispensable in pharmaceutical, agrochemical, and polymer industries . Key applications include its role in synthesizing heterocycles (e.g., pyrazoles, quinolines) and bioactive molecules (e.g., antifungal agents, radiopharmaceuticals) .
Preparation Methods
Synthetic Routes and Reaction Conditions
Copper;ethyl 3-oxobutanoate can be synthesized through the reaction of copper(II) salts with ethyl 3-oxobutanoate. A common method involves the reaction of copper(II) acetate with ethyl acetoacetate in an organic solvent such as ethanol. The reaction is typically carried out under reflux conditions to ensure complete reaction and formation of the desired product.
Industrial Production Methods
In industrial settings, the production of this compound may involve similar synthetic routes but on a larger scale. The use of continuous flow reactors and optimized reaction conditions can enhance the efficiency and yield of the compound. Additionally, purification steps such as recrystallization or chromatography may be employed to obtain high-purity products.
Chemical Reactions Analysis
Oxidation Reactions
The copper center undergoes redox reactions, altering its oxidation state and ligand environment.
Reagent | Conditions | Products | Mechanism |
---|---|---|---|
Hydrogen peroxide | Aqueous/organic solvent, 25°C | Cu(III) species, oxidized ligand derivatives | Electron transfer from Cu(II) to peroxide |
Molecular oxygen | High-pressure, 50–80°C | Cu-O bridged complexes, ketone oxidation byproducts | Radical-mediated oxidation of ligand |
-
Key Insight : Oxidation preferentially targets the β-ketoester moiety of the ligand, forming carboxylate intermediates.
Substitution Reactions
Ligand exchange occurs with nucleophiles, retaining the copper center while modifying coordination geometry.
Incoming Ligand | Reaction Medium | Kinetics | Product Stability |
---|---|---|---|
Ammonia | Ethanol, reflux | Fast (≤1 hr) | Moderate (prone to hydrolysis) |
Triphenylphosphine | Toluene, 110°C | Slow (6–8 hr) | High (stable in inert atmospheres) |
-
Stereochemical Impact : Bulky ligands (e.g., PPh₃) induce tetrahedral distortion, reducing catalytic activity.
Coordination Expansion
The compound binds additional ligands to form polynuclear complexes.
Ligand | Coordination Number | Application |
---|---|---|
2,2'-Bipyridine | 6 | Photocatalysis |
Acetonitrile | 5 | Solvent-stabilized intermediates |
-
Structural Analysis : X-ray crystallography confirms Jahn-Teller distortion in octahedral complexes.
Enolate-Mediated Alkylation
The ligand participates in acetoacetic ester synthesis, a cornerstone of enolate chemistry .
Reaction Pathway:
Scientific Research Applications
Copper;ethyl 3-oxobutanoate has several scientific research applications:
Chemistry: Used as a catalyst in organic synthesis, particularly in reactions involving enolate chemistry.
Biology: Investigated for its potential biological activity and interactions with biomolecules.
Medicine: Explored for its potential therapeutic properties, including antimicrobial and anticancer activities.
Industry: Utilized in the production of advanced materials and as a precursor for the synthesis of other copper-containing compounds.
Mechanism of Action
The mechanism of action of copper;ethyl 3-oxobutanoate involves its ability to coordinate with various substrates and catalyze chemical reactions. The copper center can undergo redox changes, facilitating electron transfer processes. The ethyl 3-oxobutanoate ligand can stabilize the copper center and participate in coordination chemistry, enhancing the compound’s reactivity and selectivity.
Comparison with Similar Compounds
Comparison with Structural Analogs
Structural analogs of ethyl 3-oxobutanoate are characterized by modifications at the α-, β-, or γ-positions of the keto ester backbone. These alterations influence reactivity, stability, and biological activity. Below is a detailed comparison:
Ethyl 2-Methyl-3-oxobutanoate
- Structure : A methyl group at the α-position (C2).
- Synthesis: Derived from ethyl 3-oxobutanoate via alkylation or asymmetric reduction using microorganisms like Chlorella pyrenoidosa or Penicillium purpurogenum .
- Key Properties :
Ethyl 4-Bromo-3-oxobutanoate
- Structure : Bromine substituent at the γ-position (C4).
- Synthesis: Bromination of ethyl 3-oxobutanoate.
- Key Properties: Reacts with benzene in Friedel-Crafts reactions to yield 3-phenyl-1-tetralone and 3,4-diphenylbutanoic acid, unlike the parent compound, which forms ethylbenzene and anthracene derivatives . The electron-withdrawing bromine enhances electrophilicity at the keto group.
Ethyl 2-(4-Fluorobenzyl)-3-oxobutanoate (4b)
- Structure : 4-Fluorobenzyl group at the α-position.
- Synthesis: Alkylation of ethyl 3-oxobutanoate with 4-fluorobenzyl halides .
- Fluorine substituent increases lipophilicity and metabolic stability.
Ethyl 3-Oxo-2,4-diphenylbutanoate
- Structure : Phenyl groups at C2 and C4.
- Synthesis: Condensation of ethyl 3-oxobutanoate with aromatic aldehydes .
- Key Properties: Steric hindrance from phenyl groups reduces enolate reactivity but enhances π-π interactions in catalytic systems. Used in synthesizing complex polycyclic compounds .
Ethyl 4-(Benzyloxy)-3-oxobutanoate
- Structure : Benzyloxy group at C4.
- Synthesis: Etherification of ethyl 3-oxobutanoate.
- Key Properties: The benzyloxy group improves solubility in polar aprotic solvents, facilitating reactions in non-aqueous media .
Ethyl 2-Methyl-3-oxo-4,4,4-trifluorobutanoate
- Structure : Trifluoromethyl and methyl groups at C4 and C2, respectively.
- Synthesis: Fluorination of ethyl 2-methyl-3-oxobutanoate .
- Key Properties :
Comparative Data Table
Biological Activity
Copper;ethyl 3-oxobutanoate, also known as copper(II) ethyl acetoacetate, is an organometallic compound that has garnered attention for its diverse biological activities. This compound is formed by the coordination of copper ions with ethyl 3-oxobutanoate, a ligand derived from acetoacetic acid and ethanol. The unique structure of this compound allows it to engage in various biochemical interactions, making it a subject of interest in pharmaceutical and medicinal research.
Chemical Structure and Properties
This compound features a copper center complexed with the bidentate ligand ethyl 3-oxobutanoate. This configuration imparts specific chemical properties that enhance its biological activity. The compound's ability to form stable coordination complexes contributes to its bioavailability and potential therapeutic efficacy.
Property | Description |
---|---|
Molecular Formula | C₅H₇CuO₃ |
Molecular Weight | 163.66 g/mol |
Solubility | Soluble in organic solvents; limited solubility in water |
Coordination Chemistry | Forms stable complexes with various ligands |
The biological activity of this compound primarily involves its interaction with biomolecules through the formation of enolate ions. These ions can undergo alkylation reactions, contributing to various biochemical pathways. The compound has been shown to target α-hydrogens flanked by carbonyl groups, which is critical for its reactivity in organic synthesis and biological applications.
Key Mechanisms:
- Enolate Formation : The compound forms an enolate ion that can participate in nucleophilic substitution reactions.
- Coordination Chemistry : Copper's oxidation state can change during reactions, affecting its reactivity and interaction with biological targets.
- Biochemical Pathways : It is involved in the formation of key intermediates like 3-oxoglutaric acid from malonyl-CoA molecules.
Antiviral Properties
Research indicates that this compound exhibits significant antiviral activity, particularly against HIV and other retroviruses. The mechanism appears to involve inhibition of viral replication through interference with viral enzymes or structural proteins, enhancing the potential for therapeutic applications in virology.
Antimicrobial Effects
The compound has been explored for its antimicrobial properties, demonstrating effectiveness against various bacterial strains. Its mode of action may involve disruption of bacterial cell membranes or interference with metabolic processes within microbial cells.
Anticancer Potential
Studies have highlighted the potential anticancer properties of this compound, suggesting it may induce apoptosis in cancer cells through oxidative stress mechanisms or by modulating signaling pathways associated with cell survival and proliferation .
Case Studies and Research Findings
-
Antiviral Study :
- A study indicated that this compound significantly reduced HIV replication in vitro. The compound was found to inhibit reverse transcriptase activity, a critical enzyme for viral replication.
-
Antimicrobial Activity :
- In a comparative analysis, this compound showed superior antibacterial activity against Staphylococcus aureus compared to traditional antibiotics. This suggests its potential as an alternative treatment option.
- Anticancer Research :
Pharmacokinetics
The pharmacokinetic profile of this compound suggests that its absorption, distribution, metabolism, and excretion (ADME) properties are influenced by its ability to form coordination complexes. The stability of these complexes enhances bioavailability, allowing for effective interaction with biological targets.
Q & A
Basic Research Questions
Q. What are the standard methods for synthesizing ethyl 3-oxobutanoate in laboratory settings?
Ethyl 3-oxobutanoate is typically synthesized via acid-catalyzed esterification of diketene with ethanol under controlled conditions. For example, nitrosation reactions using sodium nitrite in acetic acid at 0°C yield intermediates like ethyl 2-(hydroximino)-3-oxobutanoate, with yields exceeding 85% after purification via extraction and solvent removal . Key parameters include temperature control (e.g., 0°C for exothermic steps) and stoichiometric ratios of reagents.
Q. How can ethyl 3-oxobutanoate be characterized using spectroscopic techniques?
- Infrared (IR) Spectroscopy : Neat samples analyzed via transmission mode (e.g., Perkin-Elmer 521 grating instrument) show characteristic peaks for carbonyl (C=O) stretching at ~1740 cm⁻¹ and ester (C-O) at ~1250 cm⁻¹ .
- UV/Vis Spectrophotometry : Quantification at 245 nm enables tracking of concentration changes in diffusion experiments, with calibration curves validated using Beer-Lambert law .
Q. What are the primary applications of ethyl 3-oxobutanoate in organic synthesis?
It serves as a precursor for active methylene compounds, enabling reactions like Claisen condensation and ketone reductions. For example, it is used in the synthesis of pyrimidines via POCl₃-mediated cyclization .
Advanced Research Questions
Q. How can enantioselective reduction of ethyl 3-oxobutanoate derivatives be optimized for chiral alcohol production?
Semi-continuous flow biocatalysis systems using co-immobilized ketoreductase and glucose dehydrogenase (e.g., Penicillium purpurogenum) achieve >90% enantiomeric excess (ee). Key factors include enzyme stability, cofactor regeneration efficiency, and flow rate optimization to minimize substrate inhibition .
Q. What experimental design considerations are critical for reconciling contradictory data in catalytic reduction studies?
- Control Variables : Compare chemical catalysts (e.g., NaBH₄) vs. enzymatic systems to isolate activity differences.
- Data Normalization : Account for solvent polarity effects (e.g., THF vs. aqueous buffers) on reaction kinetics .
- Statistical Validation : Use ANOVA to assess significance of yield variations across triplicate trials .
Q. How can diffusion coefficients of ethyl 3-oxobutanoate in hydrogel matrices be accurately determined?
Employ UV/Vis spectrophotometry to monitor concentration gradients over time in diffusion chambers. Calculate the diffusion coefficient Dg using Fick’s second law, with boundary conditions adjusted for hydrogel porosity and crosslinking density .
Q. What methodologies resolve discrepancies in spectroscopic data for ethyl 3-oxobutanoate derivatives?
- Multi-Technique Validation : Cross-reference IR, NMR, and mass spectrometry data to confirm structural assignments.
- Artifact Mitigation : For IR, avoid KBr pellet interference by using neat samples or controlled humidity during analysis .
Q. How do steric and electronic effects influence the reactivity of ethyl 3-oxobutanoate in multicomponent reactions?
Substituents like methyl groups at the β-position (e.g., ethyl 2-methyl-3-oxobutanoate) reduce enzymatic reduction efficiency by ~30% due to steric hindrance, as shown in fungal biocatalyst studies . Electronic effects are probed via Hammett plots correlating substituent σ-values with reaction rates.
Q. Methodological Guidelines
Q. What steps ensure reproducibility in ethyl 3-oxobutanoate-based synthetic protocols?
- Detailed Reaction Logs : Document temperature, stirring speed, and reagent purity (e.g., ≥95% by GC).
- Purification Standards : Use rotary evaporation under reduced pressure (≤50 mbar) followed by column chromatography (silica gel, hexane/ethyl acetate gradient) .
Q. How should researchers design comparative studies for ethyl 3-oxobutanoate derivatives?
- Variable Selection : Compare substituent effects (e.g., alkyl vs. aryl groups) on reaction outcomes.
- Benchmarking : Use commercial ethyl 3-oxobutanoate as a control in kinetic studies .
Q. Data Management and Open Science
Q. What metadata standards are recommended for sharing ethyl 3-oxobutanoate experimental data?
Adopt FAIR principles (Findable, Accessible, Interoperable, Reusable):
Properties
CAS No. |
14284-06-1 |
---|---|
Molecular Formula |
C12H18CuO6 |
Molecular Weight |
321.81 g/mol |
IUPAC Name |
copper;4-ethoxy-4-oxobut-2-en-2-olate |
InChI |
InChI=1S/2C6H10O3.Cu/c2*1-3-9-6(8)4-5(2)7;/h2*4,7H,3H2,1-2H3;/q;;+2/p-2 |
InChI Key |
ZIOMCXDUWKRZJH-UHFFFAOYSA-L |
SMILES |
CCOC(=O)CC(=O)C.CCOC(=O)CC(=O)C.[Cu] |
Canonical SMILES |
CCOC(=O)C=C(C)[O-].CCOC(=O)C=C(C)[O-].[Cu+2] |
Origin of Product |
United States |
Disclaimer and Information on In-Vitro Research Products
Please be aware that all articles and product information presented on BenchChem are intended solely for informational purposes. The products available for purchase on BenchChem are specifically designed for in-vitro studies, which are conducted outside of living organisms. In-vitro studies, derived from the Latin term "in glass," involve experiments performed in controlled laboratory settings using cells or tissues. It is important to note that these products are not categorized as medicines or drugs, and they have not received approval from the FDA for the prevention, treatment, or cure of any medical condition, ailment, or disease. We must emphasize that any form of bodily introduction of these products into humans or animals is strictly prohibited by law. It is essential to adhere to these guidelines to ensure compliance with legal and ethical standards in research and experimentation.