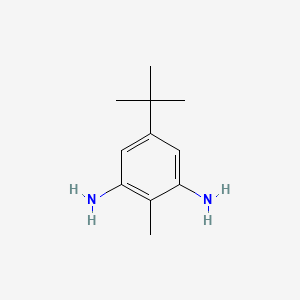
2,6-Diamino-4-t-butyltoluene
- Click on QUICK INQUIRY to receive a quote from our team of experts.
- With the quality product at a COMPETITIVE price, you can focus more on your research.
Overview
Description
2,6-Diamino-4-t-butyltoluene is a useful research compound. Its molecular formula is C11H18N2 and its molecular weight is 178.27 g/mol. The purity is usually 95%.
BenchChem offers high-quality this compound suitable for many research applications. Different packaging options are available to accommodate customers' requirements. Please inquire for more information about this compound including the price, delivery time, and more detailed information at info@benchchem.com.
Scientific Research Applications
2.1. Polyurethane Production
One of the primary applications of 2,6-Diamino-4-t-butyltoluene is in the production of polyurethane elastomers. It acts as a chain extender or crosslinking agent, enhancing the mechanical properties of the resulting polymers. The compound can be utilized in:
- Flexible and Rigid Polyurethanes : It contributes to improved elasticity and strength.
- Reaction Injection Molding (RIM) : The slower reactivity of this compound compared to other diamines makes it suitable for large automotive parts manufacturing .
Table 1: Properties of Polyurethanes Using this compound
Property | Value |
---|---|
Tensile Strength | High |
Elongation at Break | Moderate |
Hardness | Varies |
Processing Temperature | Moderate |
2.2. Synthesis of Diisocyanates
The compound is also instrumental in synthesizing diisocyanates, which are crucial precursors for polyurethanes. The reaction involves alkylation followed by nitration and reduction processes .
Case Study: Diisocyanate Production
A study demonstrated that using this compound in diisocyanate synthesis resulted in products with enhanced thermal stability and lower toxicity compared to traditional methods.
3.1. Plasticizers and Additives
This compound can serve as a plasticizer or an intermediate in producing various industrial chemicals, including:
- Pesticides : It is employed as an intermediate in synthesizing certain agricultural chemicals.
- Alkyd Resins : Used in modifying alkyd resins for improved performance characteristics .
3.2. Research Applications
In scientific research, this compound has been utilized for:
- Analytical Chemistry : It serves as a reagent in various analytical methods due to its ability to form stable complexes with metal ions.
- Material Science Studies : Investigations into its properties have led to advancements in developing new materials with tailored characteristics.
Q & A
Q. Basic: What are the established synthetic routes for 2,6-Diamino-4-t-butyltoluene, and what key reaction conditions optimize yield?
Answer:
The synthesis of this compound typically involves alkylation and amination steps. A plausible route includes:
Alkylation of toluene derivatives : Introduce the tert-butyl group at the para position via Friedel-Crafts alkylation using tert-butyl chloride and a Lewis acid catalyst (e.g., AlCl₃) under anhydrous conditions .
Directed halogenation : Bromination at the 2,6-positions using Br₂ in the presence of a directing group (e.g., nitro or acetyl) to ensure regioselectivity .
Amination : Replace halogens with amino groups via nucleophilic substitution using ammonia or amines under high-temperature/pressure conditions. Catalytic hydrogenation (e.g., Pd/C) may reduce intermediates.
Optimization : Control reaction stoichiometry, temperature, and catalyst loading to minimize side products. Purification via column chromatography or recrystallization ensures high purity .
Q. Basic: What spectroscopic and chromatographic methods are recommended for characterizing this compound?
Answer:
A multi-technique approach is critical:
- NMR Spectroscopy : ¹H/¹³C NMR identifies amine protons (δ 3.5–5.0 ppm) and tert-butyl group (δ 1.3 ppm, singlet). Aromatic protons in the 2,6-positions show distinct splitting patterns .
- Mass Spectrometry (MS) : High-resolution MS (HRMS) confirms molecular weight (e.g., [M+H]⁺ at m/z calculated for C₁₁H₁₈N₂).
- HPLC/LC-MS : Reverse-phase HPLC with UV detection (λ = 254 nm) or LC-MS assesses purity and quantifies impurities. Use C18 columns with acetonitrile/water gradients .
- Elemental Analysis : Validates C/H/N ratios (±0.3% deviation).
Q. Basic: How is this compound utilized as a building block in organic synthesis?
Answer:
Its amino and tert-butyl groups enable diverse derivatization:
- Pharmaceutical intermediates : React with acyl chlorides or sulfonyl chlorides to form amides/sulfonamides for bioactive molecule libraries .
- Ligand design : Coordinate with metals (e.g., Pd, Cu) to create catalysts for cross-coupling reactions. The tert-butyl group enhances steric hindrance, improving selectivity .
- Polymer precursors : Incorporate into fluorinated polymers via polycondensation, leveraging amine reactivity for crosslinking .
Q. Advanced: What challenges arise in crystallographic analysis of this compound, and how can they be addressed?
Answer:
Key challenges include:
- Hydrogen bonding complexity : The amino groups form intricate H-bond networks, complicating crystal packing determination. Use graph set analysis (e.g., Etter’s rules) to classify motifs like R₂²(8) rings .
- Disorder in tert-butyl groups : Large, flexible substituents cause electron density smearing. Refine using restraints in SHELXL and collect high-resolution data (<1.0 Å) .
- Polymorphism : Screen crystallization solvents (e.g., DMSO/water) to isolate stable polymorphs. Differential Scanning Calorimetry (DSC) identifies phase transitions .
Q. Advanced: How should researchers resolve contradictions in reactivity data between this compound and its derivatives?
Answer:
Contradictions often stem from:
- Steric vs. electronic effects : The tert-butyl group may sterically hinder reactions expected to proceed via electronic activation (e.g., electrophilic substitution). Quantify using DFT calculations (e.g., Mulliken charges) and kinetic studies .
- Impurity interference : Trace metals or byproducts alter reactivity. Validate purity via ICP-MS and repeat reactions under inert atmospheres .
- Solvent polarity : Solvent choice (e.g., DMF vs. THF) impacts reaction pathways. Use linear solvation energy relationships (LSERs) to model solvent effects .
Q. Advanced: What strategies enhance the biological activity of this compound derivatives in medicinal chemistry?
Answer:
- Structure-Activity Relationship (SAR) studies : Systematically modify substituents (e.g., replacing tert-butyl with CF₃) and assay against target enzymes (e.g., kinases) .
- Prodrug design : Convert amines to carbamates or Schiff bases for improved bioavailability. Hydrolyze in vivo to release active compounds .
- Toxicity mitigation : Introduce PEG chains or glucuronide moieties to reduce renal accumulation. Validate via in vitro cytotoxicity assays (e.g., HepG2 cells) .
Q. Advanced: How can researchers validate analytical methods for detecting this compound in environmental samples?
Answer:
- Solid-Phase Extraction (SPE) : Use HLB cartridges (60 mg, 3 cc) preconditioned with methanol. Elute with 2-propanol:NH₄OH (95:5) and quantify via LC-MS/MS with deuterated internal standards (e.g., 4-chloro-3-methylphenol-d2) .
- Method validation : Assess recovery (>85%), precision (RSD <10%), and LOD/LOQ (ng/L range) using spiked matrices (e.g., wastewater) .
- Cross-lab reproducibility : Collaborate with independent labs to verify results under ISO/IEC 17025 guidelines .
Preparation Methods
Alkylation of Toluenenediamine with Tertiary Olefins
The alkylation of 2,6-toluenediamine (2,6-TDA) with isobutylene represents the most industrially viable route to 2,6-TDA-4-t-BT. This method leverages Friedel-Crafts alkylation mechanisms, where the acidic catalyst facilitates electrophilic substitution at the para position relative to the methyl group on the aromatic ring . The reaction typically proceeds under elevated temperatures (160–200°C) and pressures (100–1,000 psig) to maintain the olefin in a liquid or supercritical state .
Catalytic Systems and Selectivity
Zeolitic catalysts, particularly H-Y zeolites, exhibit superior performance due to their controlled pore size and strong acid sites. As demonstrated in Example 2 of US4745223A, H-Y zeolite achieved 70% conversion of 2,6-TDA with only 3% di-alkylated byproducts . The catalyst’s pore architecture restricts the ingress of bulkier di-alkylated species, thereby enhancing selectivity. Comparative studies with montmorillonite clay and silica-alumina catalysts showed lower conversions (50–60%) and higher byproduct formation (8–15%) under identical conditions .
Table 1: Catalyst Performance in 2,6-TDA Alkylation
Catalyst | Temperature (°C) | Pressure (psig) | Conversion (%) | Mono-alkylated Product (%) | Di-alkylated Byproducts (%) |
---|---|---|---|---|---|
H-Y Zeolite | 180 | 1,100 | 70 | 67 | 3 |
Montmorillonite | 180 | 766 | 58 | 52 | 6 |
Silica-Alumina | 200 | 650 | 62 | 55 | 7 |
Reaction Mechanism and Kinetic Considerations
The alkylation proceeds via a two-step mechanism: (1) protonation of isobutylene to form a tert-butyl carbocation, and (2) electrophilic attack on the aromatic ring. The ortho-directing effect of the amino groups directs substitution to the 4-position, yielding 2,6-diamino-4-t-butyltoluene as the major product . Kinetic studies reveal a first-order dependence on toluenediamine concentration and a half-order dependence on olefin partial pressure, suggesting rate-limiting carbocation formation .
Role of Water and Acid Strength
Aqueous mineral acids (e.g., HCl, H₂SO₄) generate water as a byproduct, which deactivates Brønsted acid sites. Example 1 illustrates that 36% HCl achieved 65% conversion at 180°C but required periodic catalyst regeneration . In contrast, zeolitic systems avoid aqueous media, instead utilizing bound protons for sustained activity.
Process Optimization and Industrial Scaling
Industrial processes prioritize high space-time yields and catalyst longevity. Fixed-bed reactors operating at a liquid hourly space velocity (LHSV) of 0.5–2 h⁻¹ balance throughput and selectivity . Recycling unreacted toluenediamine and olefin improves atom economy, with pilot-scale demonstrations achieving >90% isolated yields after three cycles .
Temperature and Pressure Effects
Elevated temperatures (>180°C) accelerate reaction rates but favor thermodynamic-controlled di-alkylation. Optimal conditions identified in Example 2 (180°C, 1,100 psig) maximize mono-alkylation while suppressing side reactions . Pressure maintenance above the olefin’s critical point ensures homogeneous mixing, reducing mass transfer limitations.
Analytical Characterization and Quality Control
Gas chromatography-mass spectrometry (GC-MS) and nuclear magnetic resonance (NMR) are employed to verify product purity. Table 3 from US4745223A outlines typical impurity profiles, with <1% vicinal diamines and <5% N-alkylated isomers under optimized conditions .
Table 2: Impurity Profile of 2,6-TDA-4-t-BT
Impurity Type | Concentration (wt%) |
---|---|
Vicinal Diamines | 0.8 |
N-Alkylated Isomers | 4.2 |
Di-alkylated Products | 2.9 |
Properties
Molecular Formula |
C11H18N2 |
---|---|
Molecular Weight |
178.27 g/mol |
IUPAC Name |
5-tert-butyl-2-methylbenzene-1,3-diamine |
InChI |
InChI=1S/C11H18N2/c1-7-9(12)5-8(6-10(7)13)11(2,3)4/h5-6H,12-13H2,1-4H3 |
InChI Key |
HRXJVOPYXMBXST-UHFFFAOYSA-N |
Canonical SMILES |
CC1=C(C=C(C=C1N)C(C)(C)C)N |
Origin of Product |
United States |
Disclaimer and Information on In-Vitro Research Products
Please be aware that all articles and product information presented on BenchChem are intended solely for informational purposes. The products available for purchase on BenchChem are specifically designed for in-vitro studies, which are conducted outside of living organisms. In-vitro studies, derived from the Latin term "in glass," involve experiments performed in controlled laboratory settings using cells or tissues. It is important to note that these products are not categorized as medicines or drugs, and they have not received approval from the FDA for the prevention, treatment, or cure of any medical condition, ailment, or disease. We must emphasize that any form of bodily introduction of these products into humans or animals is strictly prohibited by law. It is essential to adhere to these guidelines to ensure compliance with legal and ethical standards in research and experimentation.