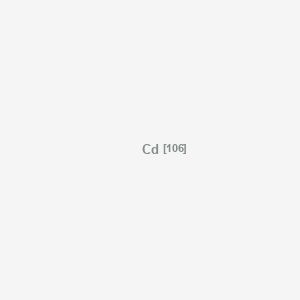
106Cd
- Click on QUICK INQUIRY to receive a quote from our team of experts.
- With the quality product at a COMPETITIVE price, you can focus more on your research.
Overview
Description
106Cd: is a stable isotope of cadmium with an atomic number of 48 and a mass number of 106. It is one of the naturally occurring isotopes of cadmium, constituting approximately 1.25% of natural cadmium . Cadmium is a soft, bluish-white metal that is chemically similar to zinc but denser and softer . It is primarily used in various industrial applications, including batteries, coatings, and pigments .
Preparation Methods
Synthetic Routes and Reaction Conditions: 106Cd can be enriched from natural cadmium using various isotopic separation techniques. One common method involves the use of gas centrifugation, where cadmium hexafluoride gas is subjected to high-speed rotation to separate isotopes based on their mass differences .
Industrial Production Methods: In industrial settings, cadmium is typically obtained as a by-product of zinc refining. The primary zinc ore, sphalerite (zinc sulfide), contains small amounts of cadmium. During the roasting process, cadmium becomes concentrated in the fumes, which are then treated to produce cadmium metal . For isotopic enrichment, specialized facilities use techniques like gas centrifugation or electromagnetic separation to isolate cadmium-106 .
Chemical Reactions Analysis
Types of Reactions: 106Cd, like other cadmium isotopes, undergoes various chemical reactions, including:
Oxidation: Cadmium reacts with oxygen to form cadmium oxide (CdO).
Reduction: Cadmium oxide can be reduced back to cadmium metal using reducing agents like hydrogen gas. [ \text{CdO} + \text{H}_2 \rightarrow \text{Cd} + \text{H}_2\text{O} ]
Substitution: Cadmium can react with halogens to form cadmium halides, such as cadmium chloride (CdCl₂) when reacting with hydrochloric acid. [ \text{Cd} + 2 \text{HCl} \rightarrow \text{CdCl}_2 + \text{H}_2 ]
Common Reagents and Conditions:
Oxidation: Oxygen or air at elevated temperatures.
Reduction: Hydrogen gas or other reducing agents at high temperatures.
Substitution: Halogen acids like hydrochloric acid, hydrobromic acid, etc.
Major Products:
- Cadmium oxide (CdO)
- Cadmium chloride (CdCl₂)
- Cadmium bromide (CdBr₂)
Scientific Research Applications
Chemistry: 106Cd is used in various chemical research applications, including the study of cadmium’s chemical properties and reactions. It is also used in the synthesis of cadmium-based compounds and materials .
Biology and Medicine: this compound is used in biological and medical research to study the effects of cadmium exposure on living organisms. It is also used in tracer studies to understand the distribution and accumulation of cadmium in biological systems .
Industry: In industrial applications, cadmium-106 is used in the production of specialized materials, such as cadmium-based semiconductors and nanomaterials. These materials have applications in electronics, photovoltaics, and catalysis .
Mechanism of Action
Molecular Targets and Pathways: Cadmium exerts its effects through various biochemical and molecular mechanisms. One primary pathway is the induction of oxidative stress, where cadmium disrupts the balance between oxidants and antioxidants, leading to cellular damage and apoptosis . Cadmium also interferes with cellular signaling pathways, such as the Mitogen-Activated Protein Kinase (MAPK) and Nuclear Factor kappa-light-chain-enhancer of activated B cells (NF-κB) pathways, contributing to pathological conditions and carcinogenesis .
Comparison with Similar Compounds
- Zinc-66: Another stable isotope of a Group 12 element, zinc-66 is chemically similar to cadmium-106 but has different physical properties and biological effects.
- Mercury-202: A stable isotope of mercury, mercury-202 shares some chemical properties with cadmium-106 but is more toxic and has different industrial applications .
Uniqueness: 106Cd is unique due to its specific isotopic properties, making it valuable for certain scientific and industrial applications. Its stable nature allows for its use in long-term studies and applications without the concerns associated with radioactive decay .
Properties
Molecular Formula |
Cd |
---|---|
Molecular Weight |
105.90646 g/mol |
IUPAC Name |
cadmium-106 |
InChI |
InChI=1S/Cd/i1-6 |
InChI Key |
BDOSMKKIYDKNTQ-VENIDDJXSA-N |
Isomeric SMILES |
[106Cd] |
Canonical SMILES |
[Cd] |
Origin of Product |
United States |
Disclaimer and Information on In-Vitro Research Products
Please be aware that all articles and product information presented on BenchChem are intended solely for informational purposes. The products available for purchase on BenchChem are specifically designed for in-vitro studies, which are conducted outside of living organisms. In-vitro studies, derived from the Latin term "in glass," involve experiments performed in controlled laboratory settings using cells or tissues. It is important to note that these products are not categorized as medicines or drugs, and they have not received approval from the FDA for the prevention, treatment, or cure of any medical condition, ailment, or disease. We must emphasize that any form of bodily introduction of these products into humans or animals is strictly prohibited by law. It is essential to adhere to these guidelines to ensure compliance with legal and ethical standards in research and experimentation.