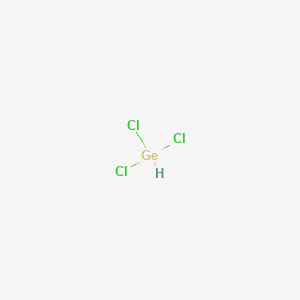
trichlorogermane
- Click on QUICK INQUIRY to receive a quote from our team of experts.
- With the quality product at a COMPETITIVE price, you can focus more on your research.
Overview
Description
Hydride Terminated Polydimethylsiloxane . This compound belongs to a group of polymeric organosilicon compounds and is widely used in various industrial applications due to its unique chemical properties .
Preparation Methods
Hydride Terminated Polydimethylsiloxane: is typically synthesized through the hydrosilylation reaction. This involves the addition of silicon-hydrogen bonds across carbon-carbon multiple bonds, usually in the presence of a platinum catalyst. The reaction conditions often include moderate temperatures and inert atmospheres to prevent unwanted side reactions . Industrial production methods involve the use of high-purity reagents and controlled environments to ensure the consistency and quality of the final product .
Chemical Reactions Analysis
Hydride Terminated Polydimethylsiloxane: undergoes several types of chemical reactions:
Oxidation: This compound can be oxidized to form silanols and siloxanes.
Reduction: It can be reduced to form silanes.
Substitution: The hydride groups can be substituted with other functional groups, such as alkyl or aryl groups, through various chemical reactions.
Common reagents used in these reactions include platinum catalysts for hydrosilylation, oxidizing agents like hydrogen peroxide for oxidation, and reducing agents like lithium aluminum hydride for reduction . The major products formed from these reactions include silanols, siloxanes, and various substituted silanes .
Scientific Research Applications
Hydride Terminated Polydimethylsiloxane: has a wide range of applications in scientific research:
Mechanism of Action
The mechanism of action of Hydride Terminated Polydimethylsiloxane involves its ability to form stable silicon-oxygen bonds. This property allows it to act as a crosslinking agent in the formation of silicone rubber and resins. The molecular targets and pathways involved include the interaction of the hydride groups with various functional groups, leading to the formation of stable siloxane bonds .
Comparison with Similar Compounds
Hydride Terminated Polydimethylsiloxane: is unique compared to other similar compounds due to its specific molecular structure and reactivity. Similar compounds include:
Polydimethylsiloxane: Lacks the hydride termination and has different reactivity and applications.
Trimethylsiloxy Terminated Polydimethylsiloxane: Has trimethylsiloxy groups instead of hydride groups, leading to different chemical properties and uses.
Vinyl Terminated Polydimethylsiloxane: Contains vinyl groups, which provide different reactivity and applications compared to hydride terminated compounds.
These comparisons highlight the unique properties and applications of Hydride Terminated Polydimethylsiloxane in various fields .
Properties
Molecular Formula |
Cl3GeH |
---|---|
Molecular Weight |
180.0 g/mol |
IUPAC Name |
trichlorogermane |
InChI |
InChI=1S/Cl3GeH/c1-4(2)3/h4H |
InChI Key |
MUDDKLJPADVVKF-UHFFFAOYSA-N |
Canonical SMILES |
Cl[GeH](Cl)Cl |
Origin of Product |
United States |
Disclaimer and Information on In-Vitro Research Products
Please be aware that all articles and product information presented on BenchChem are intended solely for informational purposes. The products available for purchase on BenchChem are specifically designed for in-vitro studies, which are conducted outside of living organisms. In-vitro studies, derived from the Latin term "in glass," involve experiments performed in controlled laboratory settings using cells or tissues. It is important to note that these products are not categorized as medicines or drugs, and they have not received approval from the FDA for the prevention, treatment, or cure of any medical condition, ailment, or disease. We must emphasize that any form of bodily introduction of these products into humans or animals is strictly prohibited by law. It is essential to adhere to these guidelines to ensure compliance with legal and ethical standards in research and experimentation.