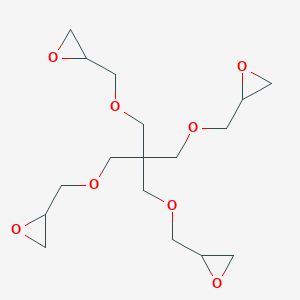
Pentaerythritol glycidyl ether
Overview
Description
Pentaerythritol glycidyl ether is an organic compound with the molecular formula C17H28O8. It is a versatile chemical used in various industrial applications due to its unique structure, which includes four glycidyl ether groups. This compound is known for its high reactivity and ability to form cross-linked networks, making it valuable in the production of coatings, adhesives, and other polymeric materials .
Preparation Methods
Synthetic Routes and Reaction Conditions
Pentaerythritol glycidyl ether is typically synthesized through the reaction of pentaerythritol with epichlorohydrin in the presence of a base such as sodium hydroxide. The reaction involves the formation of glycidyl ether groups through the substitution of hydroxyl groups on pentaerythritol with epichlorohydrin .
Industrial Production Methods
In industrial settings, the production of this compound involves large-scale batch or continuous processes. The reaction is carried out under controlled temperature and pressure conditions to ensure high yield and purity. The product is then purified through distillation or crystallization to remove any unreacted starting materials and by-products .
Chemical Reactions Analysis
Types of Reactions
Pentaerythritol glycidyl ether undergoes various chemical reactions, including:
Epoxy Ring-Opening Reactions: The glycidyl ether groups can undergo ring-opening reactions with nucleophiles such as amines, alcohols, and thiols, leading to the formation of cross-linked networks.
Polymerization: It can participate in polymerization reactions to form epoxy resins, which are used in coatings, adhesives, and composites.
Common Reagents and Conditions
Nucleophiles: Amines, alcohols, and thiols are commonly used to react with the glycidyl ether groups.
Catalysts: Lewis acids or bases are often used to catalyze the ring-opening reactions.
Conditions: Reactions are typically carried out at elevated temperatures (50-150°C) to facilitate the ring-opening process.
Major Products
The major products formed from these reactions include cross-linked polymers and epoxy resins, which have applications in coatings, adhesives, and composite materials .
Scientific Research Applications
Polymer Science
PGE is widely used as a cross-linking agent in the synthesis of advanced polymeric materials. It participates in epoxy ring-opening reactions, leading to the formation of durable cross-linked networks essential for coatings and adhesives.
Table 1: Polymer Applications of PGE
Application Type | Description |
---|---|
Coatings | Used in high-performance coatings for durability |
Adhesives | Enhances adhesion properties in various substrates |
Composites | Provides strength and stability to composite materials |
Biocompatible Materials
In the field of biology, PGE is employed in the preparation of biocompatible hydrogels . These hydrogels are crucial for drug delivery systems and tissue engineering due to their ability to mimic biological environments.
Case Study: Hydrogel Development
- Researchers developed a hydrogel using PGE that demonstrated enhanced drug release profiles compared to traditional materials, showing potential for improved therapeutic outcomes.
Medical Applications
PGE derivatives are explored for use in medical adhesives and coatings for medical devices. The compound's biocompatibility and adhesive properties make it suitable for applications requiring strong bonding without compromising safety.
Table 2: Medical Applications of PGE Derivatives
Application Type | Description |
---|---|
Medical Adhesives | Strong bonding agents for surgical applications |
Coatings | Protective coatings for implants and devices |
Industrial Uses
PGE is extensively utilized in various industrial applications, including:
- Paints and Coatings: As a surfactant and reactive diluent, it enhances the performance of appliance paints, marine coatings, and automotive finishes.
- Sealants: Provides flexibility and durability in sealants used in construction and automotive industries.
Table 3: Industrial Applications of PGE
Application Type | Description |
---|---|
Paints | Improves adhesion and durability |
Sealants | Offers flexibility and resistance to environmental factors |
Mechanism of Action
The mechanism of action of pentaerythritol glycidyl ether involves the ring-opening of the glycidyl ether groups, which then react with nucleophiles to form cross-linked networks. This cross-linking enhances the mechanical properties and chemical resistance of the resulting materials. The molecular targets include hydroxyl, amine, and thiol groups on other molecules, which participate in the ring-opening reactions .
Comparison with Similar Compounds
Similar Compounds
Neopentyl glycol diglycidyl ether: Similar in structure but with only two glycidyl ether groups.
Trimethylolpropane triglycidyl ether: Contains three glycidyl ether groups and is used in similar applications.
Uniqueness
Pentaerythritol glycidyl ether is unique due to its four glycidyl ether groups, which provide higher reactivity and the ability to form more densely cross-linked networks compared to compounds with fewer glycidyl ether groups. This makes it particularly valuable in applications requiring high-performance materials .
Biological Activity
Pentaerythritol glycidyl ether (PGE) is an organic compound characterized by its molecular formula . It is widely recognized for its versatility in industrial applications, particularly in the production of coatings, adhesives, and polymeric materials. Beyond its industrial uses, PGE has garnered attention for its biological activity, particularly in the fields of drug delivery, tissue engineering, and medical device coatings. This article explores the biological activity of PGE, including its biochemical properties, cellular effects, and safety assessments.
Chemical Structure and Properties
PGE contains four glycidyl ether groups that contribute to its high reactivity. This structure allows for various chemical reactions, including epoxy ring-opening reactions and polymerization processes. The compound is synthesized through the reaction of pentaerythritol with epichlorohydrin in the presence of a base like sodium hydroxide.
Property | Value |
---|---|
Molecular Weight | 376.39 g/mol |
Density | 1.18 g/cm³ |
Melting Point | 25-30 °C |
Boiling Point | 140-150 °C |
Solubility | Soluble in organic solvents |
Drug Delivery Systems
PGE is utilized in developing biocompatible hydrogels for drug delivery systems. These hydrogels can encapsulate therapeutic agents and release them in a controlled manner, enhancing the efficacy of treatments . Research indicates that PGE-based hydrogels exhibit favorable mechanical properties and biocompatibility, making them suitable for biomedical applications.
Tissue Engineering
In tissue engineering, PGE serves as a scaffold material due to its ability to form cross-linked networks. These networks provide structural support for cell attachment and growth. Studies have demonstrated that PGE-based scaffolds can promote cell proliferation and differentiation, essential for tissue regeneration .
Medical Device Coatings
PGE derivatives are explored for use in coatings for medical devices. These coatings enhance the biocompatibility and functionality of devices such as stents and catheters. The incorporation of PGE into these applications aims to reduce thrombogenicity and improve patient outcomes .
The primary mechanism of action for PGE involves its interaction with biomolecules within biological systems. The glycidyl ether groups can react with nucleophiles such as amines and alcohols, leading to the formation of cross-linked structures that can influence cellular behavior . Additionally, PGE has been shown to react with carbon dioxide in the presence of catalysts, producing cyclic carbonates which may further participate in biological processes .
Table 2: Summary of Mechanisms
Mechanism | Description |
---|---|
Cross-linking | Reacts with nucleophiles to form stable networks |
Carbon dioxide fixation | Produces cyclic carbonates that may engage in biological activity |
Biochemical interactions | Influences cell function through interactions with biomolecules |
In Vivo Studies
Research involving animal models has assessed the safety and biological effects of PGE. In one study, rats exposed to high concentrations of PGE exhibited signs of respiratory irritation and gastrointestinal distress . However, lower doses showed no significant adverse effects, indicating a potential therapeutic window for safe application.
Clinical Observations
Clinical observations have noted that patients exposed to epoxy resin compounds containing glycidyl ethers may experience allergic reactions. Patch testing revealed sensitization effects in a subset of patients . This highlights the importance of evaluating individual responses to PGE-containing materials in clinical settings.
Safety and Toxicology
PGE is classified as a hazardous substance due to its potential irritative effects on the respiratory system and skin sensitization properties. Studies have shown that exposure can lead to symptoms such as erythema and vesiculation upon skin contact . Therefore, safety assessments are crucial when considering PGE for biomedical applications.
Table 3: Safety Profile of this compound
Safety Concern | Description |
---|---|
Respiratory Irritation | Irritation observed at high concentrations |
Skin Sensitization | Potential for allergic reactions upon contact |
Toxicological Effects | In vivo studies indicate gastrointestinal distress at high doses |
Q & A
Basic Research Questions
Q. What spectroscopic methods are effective for confirming the successful synthesis of PEGE-based derivatives?
- Methodological Answer : Nuclear Magnetic Resonance (¹H NMR) and Infrared (IR) spectroscopy are critical. For example, ¹H NMR can identify PEGE's proton peaks (e.g., δ=1.2–3.42 ppm for PEGE and δ=4.98 ppm for hydroxyl groups in derivatives like pentaerythritol-β-cyclodextrin ether). IR spectroscopy detects characteristic epoxy ring vibrations (~910 cm⁻¹) and hydroxyl stretches (~3400 cm⁻¹) .
Q. How can researchers quantify PEGE's epoxy content for quality control in experimental batches?
- Methodological Answer : Epoxy equivalent (EE) determination via titration with hydrogen bromide (HBr) in acetic acid is standard. PEGE typically has an EE of 95–115 Gm/Eq, which correlates with cross-linking potential. Ensure anhydrous conditions to avoid side reactions .
Q. What analytical techniques are suitable for detecting trace PEGE in environmental or biological samples?
- Methodological Answer : High-Performance Liquid Chromatography (HPLC) with UV detection (for aromatic derivatives) or Gas Chromatography (GC) with flame ionization (for volatile glycidyl ethers). Amberlite XAD-7 resin is effective for air sampling, with recovery rates >80% under controlled humidity .
Q. What safety protocols are critical when handling PEGE in laboratory settings?
- Methodological Answer : Use nitrile gloves, chemical-resistant aprons, and EN 166-certified eye protection. Ensure ventilation to minimize inhalation risks. Storage at room temperature (RT) in dry conditions prevents premature polymerization .
Advanced Research Questions
Q. How can cross-linking density be optimized in PEGE-based polymer electrolytes for solid-state lithium batteries?
- Methodological Answer : Adjust the molar ratio of PEGE to 1,3-dioxolane (DOL) during in situ polymerization. Higher PEGE content increases cross-linking but may reduce ionic conductivity. Electrochemical impedance spectroscopy (EIS) can measure Li⁺ mobility, targeting 10⁻⁴ S/cm at RT .
Q. What strategies mitigate data discrepancies in toxicological assessments of PEGE and its metabolites?
- Methodological Answer : Use Ames tests for mutagenicity and in vitro cell models (e.g., HepG2) for cytotoxicity. Compare results with structurally similar glycidyl ethers (e.g., phenyl glycidyl ether, Group 2B carcinogen) while accounting for PEGE’s higher steric hindrance and reduced reactivity .
Q. How does PEGE enhance the mechanical properties of soy-protein films in sustainable packaging applications?
- Methodological Answer : PEGE acts as a covalent cross-linker between protein chains. Optimize reaction pH (9–10) and temperature (60–80°C) to maximize tensile strength. Fourier-Transform Infrared (FTIR) spectroscopy tracks the disappearance of epoxy peaks, confirming cross-linking completion .
Q. What synthetic routes enable PEGE incorporation into amphiphilic copolymers for drug delivery systems?
- Methodological Answer : Ring-opening polymerization of PEGE with poly(ethylene glycol) (PEG) or poly(lactic acid) (PLA) segments. Use catalysts like triethylamine to control epoxy ring-opening kinetics. Dynamic Light Scattering (DLS) confirms micelle formation in aqueous media .
Q. How do steric effects influence PEGE’s reactivity in hybrid material synthesis compared to smaller glycidyl ethers?
- Methodological Answer : PEGE’s tetrafunctional structure increases steric hindrance, slowing nucleophilic attacks. Kinetic studies (e.g., monitoring epoxy conversion via ¹H NMR) reveal lower reaction rates compared to monoglycidyl ethers like allyl glycidyl ether. Compensate with elevated temperatures (80–100°C) or Lewis acid catalysts .
Properties
IUPAC Name |
2-[[3-(oxiran-2-ylmethoxy)-2,2-bis(oxiran-2-ylmethoxymethyl)propoxy]methyl]oxirane | |
---|---|---|
Source | PubChem | |
URL | https://pubchem.ncbi.nlm.nih.gov | |
Description | Data deposited in or computed by PubChem | |
InChI |
InChI=1S/C17H28O8/c1(13-5-22-13)18-9-17(10-19-2-14-6-23-14,11-20-3-15-7-24-15)12-21-4-16-8-25-16/h13-16H,1-12H2 | |
Source | PubChem | |
URL | https://pubchem.ncbi.nlm.nih.gov | |
Description | Data deposited in or computed by PubChem | |
InChI Key |
PLDLPVSQYMQDBL-UHFFFAOYSA-N | |
Source | PubChem | |
URL | https://pubchem.ncbi.nlm.nih.gov | |
Description | Data deposited in or computed by PubChem | |
Canonical SMILES |
C1C(O1)COCC(COCC2CO2)(COCC3CO3)COCC4CO4 | |
Source | PubChem | |
URL | https://pubchem.ncbi.nlm.nih.gov | |
Description | Data deposited in or computed by PubChem | |
Molecular Formula |
C17H28O8 | |
Source | PubChem | |
URL | https://pubchem.ncbi.nlm.nih.gov | |
Description | Data deposited in or computed by PubChem | |
Related CAS |
171564-01-5 | |
Record name | Oxirane, 2,2′-[[2,2-bis[(oxiranylmethoxy)methyl]-1,3-propanediyl]bis(oxymethylene)]bis-, homopolymer | |
Source | CAS Common Chemistry | |
URL | https://commonchemistry.cas.org/detail?cas_rn=171564-01-5 | |
Description | CAS Common Chemistry is an open community resource for accessing chemical information. Nearly 500,000 chemical substances from CAS REGISTRY cover areas of community interest, including common and frequently regulated chemicals, and those relevant to high school and undergraduate chemistry classes. This chemical information, curated by our expert scientists, is provided in alignment with our mission as a division of the American Chemical Society. | |
Explanation | The data from CAS Common Chemistry is provided under a CC-BY-NC 4.0 license, unless otherwise stated. | |
DSSTOX Substance ID |
DTXSID10863108 | |
Record name | 2,2'-[(2,2-Bis{[(oxiran-2-yl)methoxy]methyl}propane-1,3-diyl)bis(oxymethylene)]bis(oxirane) | |
Source | EPA DSSTox | |
URL | https://comptox.epa.gov/dashboard/DTXSID10863108 | |
Description | DSSTox provides a high quality public chemistry resource for supporting improved predictive toxicology. | |
Molecular Weight |
360.4 g/mol | |
Source | PubChem | |
URL | https://pubchem.ncbi.nlm.nih.gov | |
Description | Data deposited in or computed by PubChem | |
CAS No. |
3126-63-4 | |
Record name | Pentaerythritol tetraglycidyl ether | |
Source | CAS Common Chemistry | |
URL | https://commonchemistry.cas.org/detail?cas_rn=3126-63-4 | |
Description | CAS Common Chemistry is an open community resource for accessing chemical information. Nearly 500,000 chemical substances from CAS REGISTRY cover areas of community interest, including common and frequently regulated chemicals, and those relevant to high school and undergraduate chemistry classes. This chemical information, curated by our expert scientists, is provided in alignment with our mission as a division of the American Chemical Society. | |
Explanation | The data from CAS Common Chemistry is provided under a CC-BY-NC 4.0 license, unless otherwise stated. | |
Record name | Pentaerythritol glycidyl ether | |
Source | ChemIDplus | |
URL | https://pubchem.ncbi.nlm.nih.gov/substance/?source=chemidplus&sourceid=0003126634 | |
Description | ChemIDplus is a free, web search system that provides access to the structure and nomenclature authority files used for the identification of chemical substances cited in National Library of Medicine (NLM) databases, including the TOXNET system. | |
Record name | 2,2'-[(2,2-Bis{[(oxiran-2-yl)methoxy]methyl}propane-1,3-diyl)bis(oxymethylene)]bis(oxirane) | |
Source | EPA DSSTox | |
URL | https://comptox.epa.gov/dashboard/DTXSID10863108 | |
Description | DSSTox provides a high quality public chemistry resource for supporting improved predictive toxicology. | |
Record name | 1,3-bis(2,3-epoxypropoxy)-2,2-bis[(2,3-epoxypropoxy)methyl]propane | |
Source | European Chemicals Agency (ECHA) | |
URL | https://echa.europa.eu/substance-information/-/substanceinfo/100.019.552 | |
Description | The European Chemicals Agency (ECHA) is an agency of the European Union which is the driving force among regulatory authorities in implementing the EU's groundbreaking chemicals legislation for the benefit of human health and the environment as well as for innovation and competitiveness. | |
Explanation | Use of the information, documents and data from the ECHA website is subject to the terms and conditions of this Legal Notice, and subject to other binding limitations provided for under applicable law, the information, documents and data made available on the ECHA website may be reproduced, distributed and/or used, totally or in part, for non-commercial purposes provided that ECHA is acknowledged as the source: "Source: European Chemicals Agency, http://echa.europa.eu/". Such acknowledgement must be included in each copy of the material. ECHA permits and encourages organisations and individuals to create links to the ECHA website under the following cumulative conditions: Links can only be made to webpages that provide a link to the Legal Notice page. | |
Retrosynthesis Analysis
AI-Powered Synthesis Planning: Our tool employs the Template_relevance Pistachio, Template_relevance Bkms_metabolic, Template_relevance Pistachio_ringbreaker, Template_relevance Reaxys, Template_relevance Reaxys_biocatalysis model, leveraging a vast database of chemical reactions to predict feasible synthetic routes.
One-Step Synthesis Focus: Specifically designed for one-step synthesis, it provides concise and direct routes for your target compounds, streamlining the synthesis process.
Accurate Predictions: Utilizing the extensive PISTACHIO, BKMS_METABOLIC, PISTACHIO_RINGBREAKER, REAXYS, REAXYS_BIOCATALYSIS database, our tool offers high-accuracy predictions, reflecting the latest in chemical research and data.
Strategy Settings
Precursor scoring | Relevance Heuristic |
---|---|
Min. plausibility | 0.01 |
Model | Template_relevance |
Template Set | Pistachio/Bkms_metabolic/Pistachio_ringbreaker/Reaxys/Reaxys_biocatalysis |
Top-N result to add to graph | 6 |
Feasible Synthetic Routes
Disclaimer and Information on In-Vitro Research Products
Please be aware that all articles and product information presented on BenchChem are intended solely for informational purposes. The products available for purchase on BenchChem are specifically designed for in-vitro studies, which are conducted outside of living organisms. In-vitro studies, derived from the Latin term "in glass," involve experiments performed in controlled laboratory settings using cells or tissues. It is important to note that these products are not categorized as medicines or drugs, and they have not received approval from the FDA for the prevention, treatment, or cure of any medical condition, ailment, or disease. We must emphasize that any form of bodily introduction of these products into humans or animals is strictly prohibited by law. It is essential to adhere to these guidelines to ensure compliance with legal and ethical standards in research and experimentation.