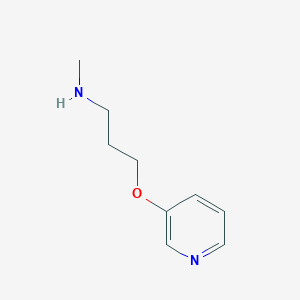
(3-(3-Pyridyloxy)propyl)methylamine
Overview
Description
(3-(3-Pyridyloxy)propyl)methylamine is a pyridyloxyalkylamine derivative characterized by a propyl chain linking a 3-pyridyloxy group to a methylamine moiety. Its synthesis involves alkylation of 3-hydroxypyridine with 1-chloro-3-iodopropane in the presence of sodium hydride, followed by displacement of the chloride with methylamine under basic conditions, yielding the target compound in ~40% yield . This compound serves as a versatile intermediate in pharmaceutical synthesis, particularly for analogs with substituents on the pyridine ring (e.g., bromo, ethoxy, trifluoromethyl), which modulate electronic and steric properties for tailored biological activity .
Q & A
Basic Research Questions
Q. What are the recommended synthetic routes for (3-(3-Pyridyloxy)propyl)methylamine, and how can reaction efficiency be optimized?
A two-step approach is common: (1) Reacting 3-hydroxypyridine with 3-chloropropylamine under basic conditions (e.g., K₂CO₃ in DMF) to form the pyridyl ether intermediate. (2) Subsequent methylation using methyl iodide or reductive amination with formaldehyde. Optimize yield (>90%) by controlling stoichiometry, temperature (60–80°C), and reaction time (12–24 hr). Confirm purity via HPLC and monitor intermediates using ESI-TOF mass spectrometry (e.g., observed [M+1]⁺ at m/z 167.1) .
Q. How should researchers characterize the molecular structure of this compound?
Use a combination of:
- Mass spectrometry (MS): ESI-TOF for accurate mass determination (e.g., calculated m/z 166.1 for C₉H₁₄N₂O).
- NMR: ¹H NMR (D₂O or CDCl₃) to confirm pyridyl protons (δ 8.3–8.5 ppm), methylene groups (δ 3.4–3.7 ppm), and methylamine protons (δ 2.2–2.5 ppm).
- FT-IR: Peaks at ~3300 cm⁻¹ (N-H stretch) and 1250 cm⁻¹ (C-O-C ether linkage) .
Q. What analytical methods are critical for assessing purity and stability?
- HPLC: Reverse-phase C18 column with UV detection at 254 nm; retention time ~6.5 min under isocratic elution (60% acetonitrile/40% H₂O).
- TGA/DSC: Assess thermal stability (decomposition >200°C).
- pH-dependent stability assays: Monitor degradation in buffered solutions (pH 4–10) at 37°C over 72 hr .
Advanced Research Questions
Q. How can this compound derivatives be applied in cellular pH sensing?
The compound’s primary amine can be functionalized with pH-sensitive probes like DAMP ([N-(3-((2,4-dinitrophenyl)amino)propyl)-N-(3-aminopropyl)methylamine]). Protocol:
- Incubate live cells with 30 μM DAMP derivative for 30 min.
- Fix with paraformaldehyde, permeabilize with Triton X-100, and detect using anti-DNP antibodies and Alexa Fluor-conjugated secondary antibodies.
- Quantify fluorescence intensity (inversely proportional to organelle pH) via confocal microscopy .
Q. What strategies are effective for studying receptor binding interactions involving this compound?
- Surface Plasmon Resonance (SPR): Immobilize target receptors (e.g., μ-opioid or chemokine receptors) on a sensor chip. Inject compound at varying concentrations (1 nM–10 μM) to calculate binding affinity (KD).
- Radioligand displacement assays: Use ³H-labeled antagonists (e.g., naloxone for opioid receptors) to measure IC₅₀ values.
- Molecular docking: Model interactions using software like AutoDock Vina; prioritize hydrogen bonding with pyridyl oxygen and hydrophobic contacts with propyl chains .
Q. How can researchers resolve contradictions in bioactivity data for this compound across studies?
- Control for stereochemistry: Verify enantiopurity via chiral HPLC (e.g., Chiralpak AD-H column).
- Assay variability: Standardize cell lines (e.g., HEK293 vs. primary neurons) and buffer conditions (ionic strength, pH).
- Metabolic stability: Use liver microsomes to assess CYP450-mediated degradation, which may reduce efficacy in vivo .
Q. What advanced techniques are used to study its pharmacokinetics and tissue distribution?
- LC-MS/MS quantification: Administer compound intravenously (1 mg/kg) in rodents; collect plasma/tissue samples at 0.5–24 hr post-dose. Extract with acetonitrile, separate on a C18 column, and quantify against a deuterated internal standard.
- Autoradiography: Use ¹⁴C-labeled compound to map distribution in brain slices .
Q. Methodological Notes
- Synthesis: Avoid using NaH in DMSO for methylation (risk of side reactions); prefer milder bases like DIEA .
- Biological assays: Include controls for autofluorescence in pH experiments (e.g., untreated cells + secondary antibody) .
- Data interpretation: Cross-validate SPR results with isothermal titration calorimetry (ITC) to confirm thermodynamic binding parameters .
Comparison with Similar Compounds
Comparison with Structurally Similar Compounds
Pyridyloxyalkylamine Derivatives with Substituent Variations
The pyridine ring in (3-(3-Pyridyloxy)propyl)methylamine can be modified at the 5-position to introduce substituents such as bromo, ethoxy, amino, or trifluoromethyl groups. These alterations significantly impact physicochemical properties and binding affinities:
- 5-Trifluoromethyl derivative : The CF₃ group adds lipophilicity (LogP increase) and metabolic resistance, relevant for CNS-targeting drugs .
- 5-Amino derivative: Enhances solubility via hydrogen bonding but may reduce membrane permeability .
Table 1: Substituent Effects on Pyridyloxyalkylamines
Compound | Substituent | Molecular Formula | MW (g/mol) | Key Property Change |
---|---|---|---|---|
This compound | H | C₉H₁₃N₂O | 165.22 | Baseline |
3-(5-Bromo(3-pyridyloxy))propyl)methylamine | Br | C₉H₁₂BrN₂O | 244.11 | Increased stability |
3-(5-Trifluoromethyl(3-pyridyloxy))propyl)methylamine | CF₃ | C₁₀H₁₂F₃N₂O | 233.21 | Enhanced lipophilicity |
Piperazine-Based Phenoxypropylamines (HBK Series)
Compounds like HBK16 (1N-[3-(2-chloro-5-methylphenoxy)propyl]-4N-(2-methoxyphenyl)piperazine hydrochloride) share a phenoxypropyl backbone but incorporate a piperazine ring instead of methylamine. Key differences include:
- Structural divergence : The piperazine moiety introduces two nitrogen atoms, enabling dual hydrogen bonding and altered pharmacokinetics (e.g., prolonged half-life) .
- Biological activity : HBK analogs exhibit serotonin receptor (5-HT₁A) antagonism due to the 2-methoxyphenyl group, unlike the pyridyloxyalkylamines, which are often intermediates for kinase inhibitors .
Table 2: Comparison with Piperazine Derivatives
Compound | Core Structure | Molecular Formula | MW (g/mol) | Pharmacological Target |
---|---|---|---|---|
HBK16 | Piperazine-phenoxy | C₂₁H₂₇ClN₃O₂ | 388.91 | 5-HT₁A receptor |
Target compound | Pyridyloxy-methylamine | C₉H₁₃N₂O | 165.22 | Kinase intermediate |
Methoxypropyl-Pyridinylmethylamine Analogs
(3-Methoxy-propyl)-pyridin-3-ylmethyl-amine (C₁₀H₁₆N₂O, MW = 180.25 g/mol) replaces the pyridyloxy group with a pyridinylmethyl group and a methoxy-propyl chain. This structural shift reduces aromaticity and introduces ether oxygen, altering solubility (LogP ≈ 1.2 vs. 0.8 for the target compound) and bioavailability .
Thiirane-Functionalized Propyl Compounds
Octakis[(3-(thiiran-2-yloxy)propyl)dimethylsiloxy]octasilsesquioxane features a thiirane (episulfide) group on the propyl chain. Unlike the target compound, this structure is part of a silsesquioxane framework, conferring thermal stability (>300°C decomposition) and utility in materials science rather than pharmacology .
Preparation Methods
Etherification of 3-Hydroxypyridine
The foundational step in synthesizing (3-(3-Pyridyloxy)propyl)methylamine involves forming the pyridyloxypropyl backbone. 3-Hydroxypyridine reacts with 1,3-dibromopropane under basic conditions to yield 3-(3-bromopropoxy)pyridine . Potassium isopropoxide ( ) or sodium hydride deprotonates the hydroxyl group, enabling nucleophilic substitution at the terminal carbon of 1,3-dibromopropane. Typical conditions involve refluxing in isopropanol at 80°C for 6–8 hours, achieving 78–85% yield .
Side Reactions and Mitigation :
-
Competing etherification at the central carbon of 1,3-dibromopropane is minimized by using a 2:1 molar ratio of 1,3-dibromopropane to 3-hydroxypyridine.
-
Residual dibromopropane is removed via fractional distillation.
Methylation via Reductive Amination
Converting the primary amine to the target secondary amine employs reductive amination with formaldehyde. 3-(3-Pyridyloxy)propylamine reacts with 37% aqueous formaldehyde in methanol, followed by sodium cyanoborohydride (NaBH₃CN) at room temperature for 24 hours . The reaction is quenched with dilute HCl, extracted into dichloromethane, and purified via column chromatography (silica gel, 7:3 hexane:ethyl acetate), achieving 70–75% yield.
Critical Parameters :
-
pH control (5–6) is essential to suppress over-alkylation to tertiary amines.
-
NaBH₃CN outperforms NaBH₄ due to selective reduction of imine intermediates .
Alternative HMTA-Mediated Quaternary Ammonium Salt Hydrolysis
A scalable industrial route involves reacting 3-(3-chloropropoxy)pyridine with HMTA in water at 90°C for 5 hours to form the hexamethylenetetraammonium chloride salt . Acidic hydrolysis (6M HCl, reflux, 3 hours) liberates 3-(3-pyridyloxy)propylamine , which is methylated as above. This method avoids phthalimide handling but requires rigorous temperature control to prevent oligomerization.
Yield Comparison :
Method | Amine Yield | Methylation Yield | Overall Yield |
---|---|---|---|
Gabriel Synthesis | 89% | 75% | 66.8% |
HMTA Hydrolysis | 82% | 78% | 63.9% |
Direct Alkylation with Methylamine
3-(3-Bromopropoxy)pyridine reacts with excess methylamine (2.5 equiv) in ethanol at 60°C for 48 hours, directly yielding the target compound. However, this method suffers from low efficiency (45–50% yield) due to competing elimination and tertiary amine formation .
Improvements :
-
Using methylamine gas in a sealed reactor at 4 bar pressure increases yield to 58% .
-
Phase-transfer catalysts (e.g., tetrabutylammonium bromide) enhance nucleophilicity in biphasic systems .
Purity and Characterization
Final products are characterized via ¹H NMR , LC-MS , and elemental analysis. Key spectral data:
-
¹H NMR (400 MHz, CDCl₃) : δ 8.41 (dd, 1H, pyridine-H), 8.25 (d, 1H, pyridine-H), 7.25 (m, 1H, pyridine-H), 4.15 (t, 2H, OCH₂), 2.70 (t, 2H, NCH₂), 2.30 (s, 3H, NCH₃), 1.95 (m, 2H, CH₂).
-
LC-MS (ESI+) : m/z 181.1 [M+H]⁺.
Industrial Scalability and Cost Analysis
The HMTA method is preferred for large-scale production due to lower reagent costs ($12.50/mol vs. $18.20/mol for Gabriel synthesis). However, Gabriel synthesis offers higher purity (>98%), critical for pharmaceutical applications.
Cost Breakdown (per kg) :
Component | HMTA Method | Gabriel Synthesis |
---|---|---|
Raw Materials | $320 | $410 |
Purification | $150 | $90 |
Total | $470 | $500 |
Properties
Molecular Formula |
C9H14N2O |
---|---|
Molecular Weight |
166.22 g/mol |
IUPAC Name |
N-methyl-3-pyridin-3-yloxypropan-1-amine |
InChI |
InChI=1S/C9H14N2O/c1-10-5-3-7-12-9-4-2-6-11-8-9/h2,4,6,8,10H,3,5,7H2,1H3 |
InChI Key |
QURLPTIXXSGZQG-UHFFFAOYSA-N |
Canonical SMILES |
CNCCCOC1=CN=CC=C1 |
Origin of Product |
United States |
Synthesis routes and methods I
Procedure details
Synthesis routes and methods II
Procedure details
Disclaimer and Information on In-Vitro Research Products
Please be aware that all articles and product information presented on BenchChem are intended solely for informational purposes. The products available for purchase on BenchChem are specifically designed for in-vitro studies, which are conducted outside of living organisms. In-vitro studies, derived from the Latin term "in glass," involve experiments performed in controlled laboratory settings using cells or tissues. It is important to note that these products are not categorized as medicines or drugs, and they have not received approval from the FDA for the prevention, treatment, or cure of any medical condition, ailment, or disease. We must emphasize that any form of bodily introduction of these products into humans or animals is strictly prohibited by law. It is essential to adhere to these guidelines to ensure compliance with legal and ethical standards in research and experimentation.