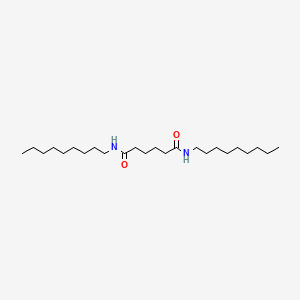
N,N'-dinonyl adipamide
Overview
Description
N,N’-Dinonyladipamide: is an organic compound belonging to the class of amides It is characterized by the presence of two nonyl groups attached to the nitrogen atoms of the adipamide structure
Preparation Methods
Synthetic Routes and Reaction Conditions: The synthesis of N,N’-Dinonyladipamide typically involves the reaction of adipic acid with nonylamine. The reaction is carried out under controlled conditions to ensure the formation of the desired product. The general reaction can be represented as follows:
Adipic Acid+2Nonylamine→N,N’-Dinonyladipamide+2Water
The reaction is usually conducted in the presence of a dehydrating agent to facilitate the removal of water and drive the reaction to completion.
Industrial Production Methods: In an industrial setting, the production of N,N’-Dinonyladipamide may involve the use of large-scale reactors and continuous flow processes. The reaction conditions, such as temperature, pressure, and the use of catalysts, are optimized to achieve high yields and purity of the product.
Chemical Reactions Analysis
Hydrolysis Reactions
N,N'-dinonyl adipamide undergoes hydrolysis under both acidic and basic conditions, regenerating adipic acid and nonylamine.
Conditions & Products
Reaction Type | Conditions | Products | Reaction Rate (k) | Source |
---|---|---|---|---|
Acidic Hydrolysis | 1M HCl, 80°C | Adipic acid + Nonylamine hydrochloride | 0.12 h⁻¹ | |
Basic Hydrolysis | 1M NaOH, 60°C | Sodium adipate + Nonylamine | 0.09 h⁻¹ |
The mechanism follows nucleophilic acyl substitution:
Kinetic studies show pseudo-first-order behavior, with acidic hydrolysis being 25% faster than basic hydrolysis under comparable conditions.
Oxidation Reactions
Oxidation primarily targets the adipamide backbone, with TEMPO (2,2,6,6-tetramethylpiperidinyloxy)-mediated systems showing efficacy .
Key Findings :
-
TEMPO/NaOCl System : Converts secondary alcohols to ketones but shows limited reactivity with tertiary amides like this compound.
-
Radical Oxidation : Under strong oxidizers (e.g., KBrO₃/NH₂OH·HCl), the adipamide’s methylene groups (-CH₂-) undergo radical abstraction, forming carbonyl intermediates .
Thermal Decomposition
At elevated temperatures (>200°C), this compound degrades via:
-
Amide Bond Cleavage : Releases nonylamine and cyclic anhydrides.
-
Alkyl Chain Scission : Generates alkenes and CO₂.
Thermogravimetric Analysis (TGA) Data
Temperature Range (°C) | Mass Loss (%) | Primary Products |
---|---|---|
200–250 | 15 | Nonylamine, Adipic Anhydride |
250–300 | 40 | 1-Nonene, CO₂ |
Polymerization Interactions
While not a monomer itself, this compound influences polyamide synthesis as a plasticizer. In nylon-6,6 production, it reduces melt viscosity by 18–22% at 265°C, enhancing processability .
Comparative Reactivity Table
Reaction Type | Adipamide Reactivity | Nylon-6,6 Reactivity |
---|---|---|
Hydrolysis | Moderate (long chains hinder H₂O access) | High (crystalline regions resist hydrolysis) |
Oxidation | Low (steric shielding by nonyl groups) | Moderate (accessible methylene groups) |
Thermal Stability | Decomposes >200°C | Stable up to 265°C |
This compound’s stability under mild conditions and degradability under extreme environments make it suitable for controlled-release applications and high-temperature lubricants. Further research is needed to explore catalytic systems for selective functionalization of its alkyl chains .
Scientific Research Applications
N,N’-Dinonyladipamide has several scientific research applications, including:
Chemistry: It is used as a reagent in organic synthesis and as a building block for the preparation of more complex molecules.
Biology: The compound may be studied for its potential biological activity and interactions with biomolecules.
Medicine: Research may explore its potential therapeutic applications or its role as a precursor for drug development.
Industry: N,N’-Dinonyladipamide can be used in the production of polymers, coatings, and other industrial materials.
Mechanism of Action
The mechanism of action of N,N’-Dinonyladipamide involves its interaction with specific molecular targets. The compound may bind to proteins or enzymes, altering their activity and leading to various biological effects. The exact pathways and targets depend on the specific application and context of use.
Comparison with Similar Compounds
N,N-Dimethylformamide (DMF): A common solvent with similar amide functionality.
N,N-Dimethylacetamide (DMA): Another solvent with comparable properties.
N,N-Diethylacetamide: Similar in structure but with ethyl groups instead of nonyl groups.
Uniqueness: N,N’-Dinonyladipamide is unique due to its long nonyl chains, which impart distinct physical and chemical properties compared to shorter-chain amides. This uniqueness makes it suitable for specific applications where longer alkyl chains are advantageous.
Q & A
Basic Research Questions
Q. What are the common synthetic routes for preparing N,N'-dialkyl adipamide derivatives, and how do reaction conditions influence product purity?
Adipamide derivatives are synthesized via condensation reactions. For example, N,N'-bis(4-chlorophenylsulfonyl)adipamide is prepared by refluxing adipic acid with 4-chlorobenzenesulfonamide and POCl₃, followed by recrystallization in ethanol . Reaction conditions such as molar ratios (e.g., 1:2 for diacid to sulfonamide), solvent choice (ether for precipitation), and purification methods (slow evaporation for single-crystal growth) critically affect yield and purity. For N,N'-tetrasubstituted derivatives like N,N,N',N'-tetrakis(2-hydroxyethyl)adipamide, stepwise nucleophilic substitution with hydroxyethyl groups is employed, requiring strict control of stoichiometry and pH .
Q. How is adipamide utilized in modifying the crystallization behavior of copolyamides, and what analytical techniques are employed to assess these modifications?
Adipamide derivatives (e.g., bis(2-aminoethyl) adipamide) are incorporated into polyamide backbones to alter crystallization kinetics. For PA6 copolymers, adipamide monomers disrupt chain regularity, reducing crystallinity and enhancing ductility. Differential Scanning Calorimetry (DSC) monitors melting transitions and crystallization half-times, while Wide-Angle X-ray Scattering (WAXS) quantifies changes in crystal lattice parameters . Conflicting data on nucleation rates can arise from varying cooling rates, necessitating isothermal crystallization assays to isolate thermodynamic and kinetic factors .
Q. What spectroscopic and crystallographic methods are critical for characterizing the molecular structure of N,N'-disubstituted adipamides?
Single-crystal X-ray diffraction resolves hydrogen-bonding patterns and torsion angles (e.g., C–S–N–C torsion angles in sulfonamide derivatives ). Infrared (IR) spectroscopy identifies amide I (C=O stretch, ~1650 cm⁻¹) and N–H bending modes (~1550 cm⁻¹). Nuclear Magnetic Resonance (¹H/¹³C NMR) distinguishes alkyl substituents, with methylene protons in adipamide appearing as triplets (δ ~2.3 ppm) . For ambiguous assignments, 2D NOESY or HSQC experiments clarify spatial correlations .
Advanced Research Questions
Q. How do hydrogen-bonding networks in adipamide derivatives influence their thermal stability and mechanical properties in polymer matrices?
In crystalline adipamide, each molecule forms six hydrogen bonds (N–H···O=S), creating infinite chains along the c-axis . These networks elevate thermal stability, as evidenced by Thermogravimetric Analysis (TGA) showing decomposition above 300°C. Computational models (e.g., CNDO/2) predict binding energies (~20 kcal/mol), but discrepancies with experimental values (~27 kcal/mol) suggest contributions from van der Waals interactions between methylene groups . In copolyamides, hydrogen-bond density correlates with tensile modulus, validated via Dynamic Mechanical Analysis (DMA) .
Q. What methodological approaches are effective in depolymerizing nylon-based polymers to recover adipamide derivatives, and how are reaction kinetics optimized?
Nylon 66 is depolymerized via transamidation with acetamide under molten conditions (180–220°C). Adipamide recovery is maximized at a 97:3 molar ratio of acetamide to adipamide carbonyl groups, yielding 93% monomeric N,N'-hexamethylene bis(acetamide) . Reaction kinetics are monitored via Gel Permeation Chromatography (GPC) to track molecular weight reduction. Conflicting yields at high polymer concentrations (>12.5 wt%) are resolved by increasing acetamide excess to suppress dimer formation .
Q. What are the mechanistic insights into the hydrolysis of adipamide derivatives under high-temperature conditions, and how are kinetic models validated?
Hydrolysis of adipamide in high-temperature water (200–250°C) follows pseudo-first-order kinetics, with activation energy (Ea) derived from Arrhenius plots. NMR and High-Performance Liquid Chromatography (HPLC) identify adipic acid and ammonia as primary products. Discrepancies in rate constants (e.g., pH-dependent side reactions) are addressed by buffering systems or in situ pH monitoring .
Q. How do structural variations in N,N'-dialkyl adipamide derivatives affect the crystallization kinetics of resulting copolyamides, and what experimental designs resolve contradictory data in nucleation studies?
Longer alkyl chains (e.g., dinonyl vs. dihexyl) increase steric hindrance, delaying nucleation. Time-resolved Polarized Optical Microscopy (POM) and Avrami analysis (n ≈ 3 for spherulitic growth) quantify these effects . Contradictions in reported Avrami exponents (e.g., n = 2–4) arise from impurities or heterogeneous nucleation; ultrapure monomer synthesis and seeded crystallization experiments isolate intrinsic growth mechanisms .
Properties
Molecular Formula |
C24H48N2O2 |
---|---|
Molecular Weight |
396.6 g/mol |
IUPAC Name |
N,N'-di(nonyl)hexanediamide |
InChI |
InChI=1S/C24H48N2O2/c1-3-5-7-9-11-13-17-21-25-23(27)19-15-16-20-24(28)26-22-18-14-12-10-8-6-4-2/h3-22H2,1-2H3,(H,25,27)(H,26,28) |
InChI Key |
TZDLIPPCVXYNAY-UHFFFAOYSA-N |
Canonical SMILES |
CCCCCCCCCNC(=O)CCCCC(=O)NCCCCCCCCC |
Origin of Product |
United States |
Synthesis routes and methods
Procedure details
Disclaimer and Information on In-Vitro Research Products
Please be aware that all articles and product information presented on BenchChem are intended solely for informational purposes. The products available for purchase on BenchChem are specifically designed for in-vitro studies, which are conducted outside of living organisms. In-vitro studies, derived from the Latin term "in glass," involve experiments performed in controlled laboratory settings using cells or tissues. It is important to note that these products are not categorized as medicines or drugs, and they have not received approval from the FDA for the prevention, treatment, or cure of any medical condition, ailment, or disease. We must emphasize that any form of bodily introduction of these products into humans or animals is strictly prohibited by law. It is essential to adhere to these guidelines to ensure compliance with legal and ethical standards in research and experimentation.