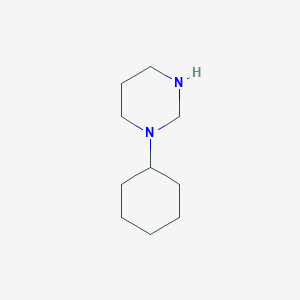
N-cyclohexyl-hexahydropyrimidine
- Click on QUICK INQUIRY to receive a quote from our team of experts.
- With the quality product at a COMPETITIVE price, you can focus more on your research.
Overview
Description
N-cyclohexyl-hexahydropyrimidine is a nitrogen-containing heterocyclic compound featuring a six-membered pyrimidine ring saturated with hydrogen atoms (hexahydropyrimidine core) and a cyclohexyl substituent attached to the nitrogen atom. Hexahydropyrimidine derivatives are structurally analogous to piperidines but with two nitrogen atoms in the ring, conferring unique electronic and steric properties.
Q & A
Q. Basic Research FAQs
Q. What are the standard synthetic routes for N-cyclohexyl-hexahydropyrimidine, and how can purity be optimized?
this compound is typically synthesized via multi-step reactions involving cyclohexylamine and carbonyl precursors. A common method includes:
- Cyclocondensation : Reacting cyclohexylamine with aldehydes or ketones under acidic or basic conditions to form the hexahydropyrimidine ring .
- Solvent selection : Polar aprotic solvents (e.g., DMF) improve reaction homogeneity, while controlled temperatures (60–80°C) prevent side reactions .
- Purification : Column chromatography (silica gel, ethyl acetate/hexane) or recrystallization (ethanol/water) achieves >95% purity. Analytical validation via 1H/13C NMR and HPLC-MS is critical .
Q. How should researchers characterize the structural and physicochemical properties of this compound?
- Spectroscopic analysis :
- Thermal stability : TGA/DSC under nitrogen (heating rate: 10°C/min) reveals decomposition temperatures (typically >200°C) .
- Solubility : Test in DMSO, ethanol, and water; logP values (e.g., via shake-flask method) guide formulation studies .
Q. What pharmacological screening strategies are recommended for initial bioactivity assessment?
- In vitro assays :
- Dose-response curves : Fit data to Hill equation for EC50/IC50 determination. Include positive controls (e.g., staurosporine for kinase inhibition) .
Q. Advanced Research FAQs
Q. How can contradictory bioactivity data between studies be resolved?
- Troubleshooting steps :
- Case example : Discrepancies in IC50 values for kinase inhibition may arise from differing ATP concentrations; standardize at 1 mM ATP .
Q. What computational methods aid in rational design of this compound derivatives?
- Molecular docking : Use AutoDock Vina to predict binding poses against target proteins (e.g., COX-2). Prioritize derivatives with ΔG < −8 kcal/mol .
- QSAR modeling : Train models on datasets with >50 analogs; descriptors include logP, molar refractivity, and H-bond donors .
- MD simulations : Run 100-ns trajectories (AMBER force field) to assess conformational stability in aqueous/lipid bilayers .
Q. How can reaction scalability challenges be addressed without compromising yield?
- Process optimization :
- Data-driven approach : Use machine learning (e.g., LabMate.AI ) to predict optimal solvent/catalyst pairs from historical reaction datasets .
Q. What strategies validate target engagement in cellular models?
- Pull-down assays : Functionalize this compound with biotin tags; use streptavidin beads to isolate protein complexes for LC-MS/MS identification .
- Cellular thermal shift assay (CETSA) : Monitor target protein melting curves after compound treatment to confirm stabilization .
- CRISPR knockouts : Compare bioactivity in wild-type vs. target gene KO cells to establish mechanism .
Comparison with Similar Compounds
The following analysis compares N-cyclohexyl-hexahydropyrimidine with structurally related compounds, focusing on synthesis, substituent effects, and functional properties.
N-(carboxymethyl)cycloheximide
- Structure : Features a carboxymethyl group instead of cyclohexyl, attached to the hexahydropyrimidine core.
- Synthesis : Synthesized via a Zn(II)-mediated reaction with cyclohexamine, achieving yields of 41.4–76.7% after preparative HPLC purification. The reaction involves PyBOP coupling agents in DMF, highlighting the influence of polar aprotic solvents on reactivity .
- Key Differences : The carboxymethyl group introduces polarity, enhancing solubility in aqueous media compared to the hydrophobic cyclohexyl group. However, steric hindrance from the cyclohexyl substituent may improve metabolic stability in biological systems.
2-(N-Butyl-hexahydropyrimidine)isoindoline-1,3-dione
- Structure : Incorporates a butyl chain and an isoindoline-1,3-dione moiety.
- Synthesis: Prepared by reacting N-(4-aminobutyl)hexahydropyrimidine with phthalimide, a protecting group strategy for primary amines. Characterized via HR-ESI-MS and NMR, demonstrating the robustness of this method for alkyl-substituted derivatives .
- Key Differences : The butyl group offers moderate hydrophobicity, intermediate between carboxymethyl and cyclohexyl. This balance may optimize membrane permeability in drug delivery applications.
Adamantane- and Aryl-Substituted Hexahydropyrimidine Amides
- Structure : Complex amides with adamantane or aryl groups (e.g., 3,5-dimethyl-1-adamantamine) linked to the hexahydropyrimidine core .
- Synthesis : Utilizes advanced coupling reagents (e.g., PyBOP) and chiral auxiliaries, yielding stereochemically defined products.
- Key Differences : Bulky adamantane groups enhance rigidity and binding affinity to hydrophobic enzyme pockets, whereas cyclohexyl groups may provide similar effects but with greater conformational flexibility.
Critical Analysis of Substituent Effects
- Cyclohexyl vs. Carboxymethyl : The cyclohexyl group’s hydrophobicity likely improves blood-brain barrier penetration compared to carboxymethyl derivatives, which are more suited for hydrophilic environments .
- Butyl vs.
- Adamantane vs. Cyclohexyl : Adamantane’s rigid structure may enhance binding to rigid enzyme pockets (e.g., viral proteases), whereas cyclohexyl’s flexibility could benefit allosteric modulation .
Properties
Molecular Formula |
C10H20N2 |
---|---|
Molecular Weight |
168.28 g/mol |
IUPAC Name |
1-cyclohexyl-1,3-diazinane |
InChI |
InChI=1S/C10H20N2/c1-2-5-10(6-3-1)12-8-4-7-11-9-12/h10-11H,1-9H2 |
InChI Key |
ZIGRSHCUYSBWPJ-UHFFFAOYSA-N |
Canonical SMILES |
C1CCC(CC1)N2CCCNC2 |
Origin of Product |
United States |
Synthesis routes and methods
Procedure details
Disclaimer and Information on In-Vitro Research Products
Please be aware that all articles and product information presented on BenchChem are intended solely for informational purposes. The products available for purchase on BenchChem are specifically designed for in-vitro studies, which are conducted outside of living organisms. In-vitro studies, derived from the Latin term "in glass," involve experiments performed in controlled laboratory settings using cells or tissues. It is important to note that these products are not categorized as medicines or drugs, and they have not received approval from the FDA for the prevention, treatment, or cure of any medical condition, ailment, or disease. We must emphasize that any form of bodily introduction of these products into humans or animals is strictly prohibited by law. It is essential to adhere to these guidelines to ensure compliance with legal and ethical standards in research and experimentation.