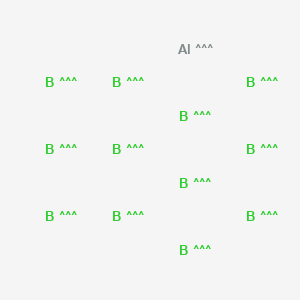
Aluminum boride
Overview
Description
Aluminum boride is an intermetallic compound consisting of aluminum and boron. It is known for its unique properties, such as high hardness, thermal stability, and resistance to wear. This compound exists in two primary forms: aluminum diboride (AlB2) and aluminum dodecaboride (AlB12). The structure of this compound involves boron atoms forming graphite-like sheets with aluminum atoms positioned between them .
Preparation Methods
Synthetic Routes and Reaction Conditions: Aluminum boride can be synthesized through various methods, including:
Direct Synthesis from Elements: This involves reacting aluminum and boron powders at high temperatures. The reaction typically occurs at temperatures above 900°C.
Mechanical Alloying: Aluminum and boron powders are mechanically alloyed and then annealed at temperatures around 650°C to form this compound.
Electrolysis of Molten Media: This method involves the electrolysis of aluminum and boron compounds in molten salts.
Carbothermal Reduction: This involves reducing aluminum oxide with boron oxide and carbon at temperatures above 1400°C.
Industrial Production Methods: Industrial production of this compound often employs high-temperature synthesis methods, such as carbothermal reduction and direct synthesis from elements. These methods are energy-intensive and require specialized equipment to maintain the necessary reaction conditions .
Chemical Reactions Analysis
Types of Reactions: Aluminum boride undergoes various chemical reactions, including:
Oxidation: this compound can oxidize in the presence of oxygen at elevated temperatures, forming aluminum oxide and boron oxide.
Reduction: It can be reduced by hydrogen or other reducing agents to form elemental aluminum and boron.
Substitution: this compound can participate in substitution reactions with other metal halides to form different metal borides.
Common Reagents and Conditions:
Oxidation: Typically occurs at high temperatures in the presence of oxygen.
Reduction: Hydrogen gas or other reducing agents are used at elevated temperatures.
Substitution: Metal halides and this compound react at high temperatures to form new metal borides.
Major Products:
Oxidation: Aluminum oxide and boron oxide.
Reduction: Elemental aluminum and boron.
Substitution: Various metal borides depending on the metal halide used.
Scientific Research Applications
Aluminum boride has numerous applications in scientific research and industry:
Catalysis: It is used as a catalyst in various chemical reactions due to its high surface area and reactivity.
Materials Science: this compound is used to enhance the mechanical properties of materials, such as hardness and wear resistance.
Electrocatalysis: It is employed in electrocatalytic applications, including hydrogen evolution reactions and nitrogen reduction reactions.
Coatings: this compound is used in protective coatings for its thermal stability and resistance to wear.
Mechanism of Action
The mechanism of action of aluminum boride in catalytic and electrocatalytic applications involves its ability to modulate local electronic structures and surface adsorption properties. The boron atoms in this compound play a crucial role in tuning the electrocatalytic performance by interacting with reactant molecules and facilitating electron transfer processes . The unique crystal structure of this compound exposes active sites that enhance its catalytic activity .
Comparison with Similar Compounds
Magnesium diboride (MgB2): Similar structure with boron atoms forming graphite-like sheets and magnesium atoms between them.
Titanium boride (TiB2): Known for its high hardness and thermal stability, used in cutting tools and wear-resistant coatings.
Zirconium boride (ZrB2): Exhibits high melting point and thermal conductivity, used in high-temperature applications.
Uniqueness of Aluminum Boride: this compound is unique due to its combination of high hardness, thermal stability, and resistance to wear. Its ability to form stable intermetallic compounds with boron and its versatility in various applications, from catalysis to protective coatings, make it a valuable material in scientific research and industry .
Biological Activity
Aluminum boride (AlB) is a compound of significant interest not only for its industrial applications but also for its potential biological activities. This article explores the biological implications of this compound, focusing on its interactions with biological systems, mechanisms of action, and potential therapeutic applications.
Overview of this compound
This compound is a binary compound formed from aluminum and boron. It has garnered attention due to its unique properties, including high thermal stability and reactivity. Recent studies have begun to investigate its biological effects, particularly in relation to aluminum toxicity and the protective roles that boron compounds can play.
Antioxidant Properties
Research indicates that aluminum exposure can lead to oxidative stress, resulting in neurotoxic and hematotoxic damage. Boron compounds, including this compound, have been shown to exhibit antioxidant properties that may mitigate these effects. For instance, studies have demonstrated that boron compounds can enhance the activity of antioxidant enzymes such as superoxide dismutase (SOD) and catalase (CAT), thereby reducing oxidative damage in cells exposed to aluminum chloride (AlCl) .
Cytoprotective Effects
In vitro studies involving primary rat cortical neurons have shown that this compound can exert cytoprotective effects against aluminum-induced toxicity. The co-application of this compound with AlCl resulted in improved cell viability compared to control groups treated with AlCl alone. This suggests that this compound may help preserve cellular integrity in the presence of toxic aluminum levels .
Case Study: Neuroprotective Effects
A study investigated the neuroprotective effects of boron compounds against aluminum-induced neurotoxicity using rat models. The results indicated significant reductions in DNA damage and oxidative stress markers in animals treated with boron compounds alongside aluminum chloride. Histopathological evaluations revealed less severe neuronal damage in treated groups compared to those exposed solely to aluminum .
Parameter | Control (AlCl₃) | AlCl₃ + this compound |
---|---|---|
DNA Damage (Comet Assay) | High | Low |
SOD Activity | Decreased | Increased |
Neuronal Integrity | Compromised | Preserved |
Case Study: Hematotoxicity Mitigation
Another investigation focused on the hematotoxic effects of aluminum exposure. In this study, human blood cultures were treated with AlCl and various concentrations of this compound. The results showed a dose-dependent protective effect where higher concentrations of this compound significantly reduced hemolysis and DNA fragmentation in blood cells exposed to aluminum .
Applications in Medicine
The potential medical applications of this compound are promising, particularly in treating conditions associated with aluminum toxicity. Given its protective effects against oxidative stress and cellular damage, there is a growing interest in developing formulations that incorporate this compound for therapeutic use.
Wound Healing Applications
Recent research has proposed using reactive metal boride nanoparticles, including this compound, as agents for wound healing. These nanoparticles can trap bacterial lipopolysaccharides (LPS), reducing inflammation while promoting healing processes. This dual action addresses both infection control and inflammatory responses, highlighting the versatility of this compound in biomedical applications .
Properties
InChI |
InChI=1S/Al.12B | |
---|---|---|
Source | PubChem | |
URL | https://pubchem.ncbi.nlm.nih.gov | |
Description | Data deposited in or computed by PubChem | |
InChI Key |
JXOOCQBAIRXOGG-UHFFFAOYSA-N | |
Source | PubChem | |
URL | https://pubchem.ncbi.nlm.nih.gov | |
Description | Data deposited in or computed by PubChem | |
Canonical SMILES |
[B].[B].[B].[B].[B].[B].[B].[B].[B].[B].[B].[B].[Al] | |
Source | PubChem | |
URL | https://pubchem.ncbi.nlm.nih.gov | |
Description | Data deposited in or computed by PubChem | |
Molecular Formula |
AlB12 | |
Source | PubChem | |
URL | https://pubchem.ncbi.nlm.nih.gov | |
Description | Data deposited in or computed by PubChem | |
DSSTOX Substance ID |
DTXSID701015528 | |
Record name | Aluminium dodecaboride | |
Source | EPA DSSTox | |
URL | https://comptox.epa.gov/dashboard/DTXSID701015528 | |
Description | DSSTox provides a high quality public chemistry resource for supporting improved predictive toxicology. | |
Molecular Weight |
156.7 g/mol | |
Source | PubChem | |
URL | https://pubchem.ncbi.nlm.nih.gov | |
Description | Data deposited in or computed by PubChem | |
CAS No. |
12041-54-2, 37367-77-4 | |
Record name | Aluminum boride (AlB12) | |
Source | CAS Common Chemistry | |
URL | https://commonchemistry.cas.org/detail?cas_rn=12041-54-2 | |
Description | CAS Common Chemistry is an open community resource for accessing chemical information. Nearly 500,000 chemical substances from CAS REGISTRY cover areas of community interest, including common and frequently regulated chemicals, and those relevant to high school and undergraduate chemistry classes. This chemical information, curated by our expert scientists, is provided in alignment with our mission as a division of the American Chemical Society. | |
Explanation | The data from CAS Common Chemistry is provided under a CC-BY-NC 4.0 license, unless otherwise stated. | |
Record name | Aluminum boride (AlB12) | |
Source | EPA Chemicals under the TSCA | |
URL | https://www.epa.gov/chemicals-under-tsca | |
Description | EPA Chemicals under the Toxic Substances Control Act (TSCA) collection contains information on chemicals and their regulations under TSCA, including non-confidential content from the TSCA Chemical Substance Inventory and Chemical Data Reporting. | |
Record name | Aluminium dodecaboride | |
Source | EPA DSSTox | |
URL | https://comptox.epa.gov/dashboard/DTXSID701015528 | |
Description | DSSTox provides a high quality public chemistry resource for supporting improved predictive toxicology. | |
Record name | Aluminium dodecaboride | |
Source | European Chemicals Agency (ECHA) | |
URL | https://echa.europa.eu/substance-information/-/substanceinfo/100.031.737 | |
Description | The European Chemicals Agency (ECHA) is an agency of the European Union which is the driving force among regulatory authorities in implementing the EU's groundbreaking chemicals legislation for the benefit of human health and the environment as well as for innovation and competitiveness. | |
Explanation | Use of the information, documents and data from the ECHA website is subject to the terms and conditions of this Legal Notice, and subject to other binding limitations provided for under applicable law, the information, documents and data made available on the ECHA website may be reproduced, distributed and/or used, totally or in part, for non-commercial purposes provided that ECHA is acknowledged as the source: "Source: European Chemicals Agency, http://echa.europa.eu/". Such acknowledgement must be included in each copy of the material. ECHA permits and encourages organisations and individuals to create links to the ECHA website under the following cumulative conditions: Links can only be made to webpages that provide a link to the Legal Notice page. | |
Record name | Aluminum boride | |
Source | European Chemicals Agency (ECHA) | |
URL | https://echa.europa.eu/substance-information/-/substanceinfo/100.048.606 | |
Description | The European Chemicals Agency (ECHA) is an agency of the European Union which is the driving force among regulatory authorities in implementing the EU's groundbreaking chemicals legislation for the benefit of human health and the environment as well as for innovation and competitiveness. | |
Explanation | Use of the information, documents and data from the ECHA website is subject to the terms and conditions of this Legal Notice, and subject to other binding limitations provided for under applicable law, the information, documents and data made available on the ECHA website may be reproduced, distributed and/or used, totally or in part, for non-commercial purposes provided that ECHA is acknowledged as the source: "Source: European Chemicals Agency, http://echa.europa.eu/". Such acknowledgement must be included in each copy of the material. ECHA permits and encourages organisations and individuals to create links to the ECHA website under the following cumulative conditions: Links can only be made to webpages that provide a link to the Legal Notice page. | |
Synthesis routes and methods
Procedure details
Disclaimer and Information on In-Vitro Research Products
Please be aware that all articles and product information presented on BenchChem are intended solely for informational purposes. The products available for purchase on BenchChem are specifically designed for in-vitro studies, which are conducted outside of living organisms. In-vitro studies, derived from the Latin term "in glass," involve experiments performed in controlled laboratory settings using cells or tissues. It is important to note that these products are not categorized as medicines or drugs, and they have not received approval from the FDA for the prevention, treatment, or cure of any medical condition, ailment, or disease. We must emphasize that any form of bodily introduction of these products into humans or animals is strictly prohibited by law. It is essential to adhere to these guidelines to ensure compliance with legal and ethical standards in research and experimentation.