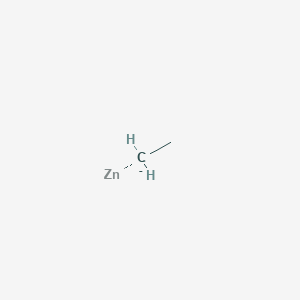
Ethylzinc
- Click on QUICK INQUIRY to receive a quote from our team of experts.
- With the quality product at a COMPETITIVE price, you can focus more on your research.
Overview
Description
Ethylzinc compounds, organometallic species containing zinc-ethyl (Zn–Et) bonds, are pivotal in synthetic chemistry for their roles in catalysis, polymer synthesis, and materials science. These compounds exhibit diverse structural motifs and reactivity profiles, influenced by ligand environments and coordination geometries . This compound derivatives, such as this compound carboxylates, amides, and guanidinates, are widely studied for their ability to form stable complexes and mediate transformations like enantioselective additions, polymerizations, and hydroaminations . Their unique balance of reactivity and stability makes them versatile compared to other organozinc species.
Preparation Methods
Synthetic Routes and Reaction Conditions
Ethane can be synthesized by the reduction of ethyl iodide using a zinc-copper couple in alcohol. The chemical equation for this reaction is: [ \text{CH₃CH₂I} + 2[\text{H}] \rightarrow \text{C₂H₆} + \text{HI} ] Another method involves the Wurtz reaction, where methyl bromide or methyl iodide reacts with sodium in the presence of dry ether to form ethane .
Industrial Production Methods
In industrial settings, ethane is primarily obtained from natural gas through processes such as fractional distillation and cryogenic separation. Zinc-containing catalysts, such as zinc zeolites, are synthesized using hydrothermal methods. For example, a Zn-embedded HZSM-5 crystal catalyst can be prepared by hydrothermal synthesis using preformed Zn/SiO₂ as the silicone source .
Chemical Reactions Analysis
Types of Reactions
Ethylzinc undergoes various chemical reactions, including:
Dehydrogenation: Ethane can be dehydrogenated to form ethylene and hydrogen.
Aromatization: Ethane can be converted to aromatic compounds such as benzene and toluene using zinc-containing zeolites
Common Reagents and Conditions
Dehydrogenation: Typically conducted at high temperatures (around 773 K) and atmospheric pressure using zinc-containing zeolites as catalysts.
Aromatization: Zinc species such as Zn²⁺ and [Zn–O–Zn]²⁺ dimers are active sites for ethane aromatization.
Major Products
Dehydrogenation: Ethylene (C₂H₄) and hydrogen (H₂).
Aromatization: Aromatic compounds such as benzene (C₆H₆) and toluene (C₇H₈)
Scientific Research Applications
Organic Synthesis
Ethylzinc as a Reagent
Dithis compound is widely utilized in organic synthesis as a source of the ethyl carbanion. Its reactivity allows for the asymmetric addition of ethyl groups to carbonyl compounds, such as benzaldehyde and imines, facilitating the formation of alcohols and amines. This property is particularly valuable in the synthesis of complex organic molecules.
Case Study: Asymmetric Synthesis
A notable application is in the asymmetric addition of dithis compound to carbonyl compounds, which has been shown to yield high enantiomeric excesses. For example, reactions involving dithis compound and imines have demonstrated efficiency in producing chiral amines with significant yields and selectivity .
Materials Science
Thin Film Deposition
Dithis compound serves as a precursor for the deposition of zinc oxide (ZnO) thin films via chemical vapor deposition (CVD) methods. The ability to form ZnO thin films is crucial for applications in electronics, optoelectronics, and photovoltaics.
Data Table: Properties of ZnO Thin Films from Dithis compound
Property | Value |
---|---|
Growth Temperature | 200-400 °C |
Film Thickness | 50-500 nm |
Resistivity | 10^-3 to 10^5 Ω·cm |
Optical Transparency | >80% at visible spectrum |
Case Study: Photovoltaic Applications
Research has shown that ZnO films produced from dithis compound exhibit excellent electrical and optical properties suitable for photovoltaic applications. The stability and efficiency of these films make them ideal for use in solar cells .
Nanotechnology
Synthesis of Nanoparticles
Dithis compound is employed in the synthesis of zinc sulfide nanoparticles, which are essential for developing core/shell-type quantum dots. These nanoparticles have unique optical properties that are exploited in various applications, including displays and biological imaging.
Data Table: Characteristics of Zinc Sulfide Nanoparticles
Parameter | Value |
---|---|
Average Size | 5-20 nm |
Zeta Potential | -30 to -50 mV |
Photoluminescence Quantum Yield | Up to 30% |
Case Study: Quantum Dot Applications
Zinc sulfide nanoparticles synthesized using dithis compound have been incorporated into quantum dot systems that demonstrate enhanced photoluminescence properties, making them suitable for use in advanced display technologies .
Industrial Applications
Corrosion Protection
In industrial settings, dithis compound is used as a corrosion-resistant coating material. Its application in galvanization processes protects metals from oxidation and extends their lifespan.
Case Study: Galvanization Process Efficiency
Studies indicate that coatings applied using dithis compound significantly reduce corrosion rates compared to untreated metals. This application is particularly beneficial in industries such as automotive manufacturing and construction .
Mechanism of Action
The mechanism of action of Ethylzinc in catalytic processes involves the activation of ethane molecules at zinc sites within the zeolite structure. Zinc species such as Zn²⁺ and [Zn–O–Zn]²⁺ dimers facilitate the dehydrogenation and aromatization reactions. The presence of Brønsted acid sites in the zeolite enhances the catalytic activity by providing additional sites for proton transfer and stabilization of reaction intermediates .
Comparison with Similar Compounds
Structural Diversity and Coordination Chemistry
Ethylzinc compounds display remarkable structural flexibility. For example, this compound carboxylates derived from monofunctional carboxylic acids (e.g., triphenylacetic acid) form dimers or 1D coordination polymers in THF, while bifunctional acids (e.g., methoxyacetic acid) yield hexanuclear, butterfly-like frameworks . In contrast, mthis compound carboxylates under similar conditions favor simpler oligomeric structures due to reduced steric bulk .
This compound amidinates, such as [Et₂Zn₃(dipf)₄], undergo Schlenk equilibria-mediated ligand scrambling, forming thermodynamically stable non-stoichiometric clusters. This contrasts with this compound pyrazolates, which retain monomeric structures in solution and only reorganize during crystallization .
Table 1: Structural Comparison of this compound and Mthis compound Derivatives
Table 2: Catalytic Performance of this compound vs. Dithis compound
Stability and Reactivity
This compound complexes exhibit enhanced steric protection in macrocyclic ligands (e.g., calix[3]-based systems), suppressing hydrolysis and alcoholysis even in aqueous environments . Conversely, this compound carboxylates are prone to ligand exchange in donor solvents like THF, forming solvated intermediates . In contrast, dimthis compound derivatives are more hydrolytically sensitive due to smaller alkyl groups and weaker Zn–C bonds .
Unique Reactivity Profiles
Similarly, this compound-mediated synthesis of fluorinated epoxides proceeds via zinc alkoxide intermediates activated by this compound aminoethoxides, a mechanism absent in bulkier organozinc reagents .
Q & A
Basic Research Questions
Q. What are the standard synthetic protocols for preparing ethylzinc complexes with tailored reactivity?
this compound complexes are typically synthesized via ligand-exchange reactions or direct alkylation. For example, ion-like this compound(II) compounds with weakly coordinating aluminates (e.g., [Al(ORᶠ)₄]⁻) are prepared via a one-pot reaction involving ZnEt₂ and fluorinated alcohols, followed by crystallization in non-polar solvents . Characterization requires single-crystal X-ray diffraction for structural elucidation, complemented by ¹H/¹³C NMR and vibrational spectroscopy to confirm ligand coordination and purity .
Q. Which spectroscopic techniques are critical for characterizing this compound intermediates in catalytic systems?
Multinuclear NMR (e.g., ¹H, ¹³C, ⁶⁷Zn) is essential for tracking ligand dynamics and Schlenk equilibria in solution . Diffuse Reflectance Infrared Fourier Transform (DRIFT) spectroscopy and X-ray absorption spectroscopy (XAS) are used to study solid-state structures and electronic environments. For example, DOSY NMR can distinguish between monomeric and oligomeric species in post-reaction mixtures .
Q. How do ligand steric and electronic properties influence this compound complex stability?
Bulky ligands (e.g., C(CF₃)₃) enhance stability by reducing ligand scrambling, while electron-withdrawing groups (e.g., fluorinated alkoxides) modulate Lewis acidity. Studies on benzamidinate ligands show that N-substituents dictate aggregation states, with steric hindrance favoring discrete [R₃Zn₄(bza)₅] clusters over polymeric structures .
Advanced Research Questions
Q. What experimental strategies optimize enantioselectivity in this compound-mediated catalytic reactions?
Enantioselectivity in alkynylation or hydroamination reactions depends on ligand design and pre-activation protocols. For instance, preforming chiral amino alcohol catalysts (e.g., N-methylephedrine lithium salts) with this compound alkoxides improves stereochemical control, as seen in phenylacetylene additions to aldehydes (up to 81% yield, 77% e.e.) . Kinetic studies under inert atmospheres are critical to minimize side reactions .
Q. How can researchers resolve contradictions in reported enantioselectivity outcomes of this compound-mediated catalytic reactions?
Contradictions often arise from competing coordination modes of multi-dentate ligands. For example, in amino diols like 18b and 18c, only one hydroxyl group participates in the active catalytic center, as shown by comparative NMR and X-ray analyses . Systematic variation of reaction conditions (temperature, solvent polarity) and isotopic labeling (e.g., deuterated substrates) can isolate contributing factors .
Q. What computational methods validate mechanistic pathways in this compound-catalyzed reactions?
Density Functional Theory (DFT) calculations are used to model transition states and identify rate-determining steps. For hydroamination reactions, studies on [EtZn(arene)₂]⁺ intermediates reveal that π-arene interactions lower activation barriers by stabilizing cationic zinc centers . Experimental validation via in situ IR spectroscopy and kinetic isotope effects (KIEs) is recommended .
Q. How do non-stoichiometric byproducts impact catalytic efficiency in this compound systems?
Schlenk equilibria often generate ZnR₂ or [RZnL]ₓ species, which compete with active catalysts. For example, in benzamidinate systems, minor products like [Me₄Zn₅(bza)₆] reduce catalytic turnover by sequestering active zinc centers. Quantitative ¹H NMR and mass spectrometry are used to monitor equilibria and quantify byproduct formation .
Q. Methodological Guidelines for Data Analysis
Q. How should researchers design experiments to account for ligand scrambling in this compound complexes?
- Use low-temperature NMR (< –30°C) to "freeze" dynamic equilibria .
- Employ chelating ligands (e.g., diphenylformamidinates) to suppress scrambling .
- Compare reactivity across ligand families (e.g., amino alcohols vs. thiolates) to isolate steric/electronic effects .
Q. What statistical approaches are suitable for analyzing contradictory data in this compound reaction kinetics?
Properties
Molecular Formula |
C2H5Zn- |
---|---|
Molecular Weight |
94.4 g/mol |
IUPAC Name |
ethane;zinc |
InChI |
InChI=1S/C2H5.Zn/c1-2;/h1H2,2H3;/q-1; |
InChI Key |
HIRPBEGJCGOCKI-UHFFFAOYSA-N |
Canonical SMILES |
C[CH2-].[Zn] |
Origin of Product |
United States |
Disclaimer and Information on In-Vitro Research Products
Please be aware that all articles and product information presented on BenchChem are intended solely for informational purposes. The products available for purchase on BenchChem are specifically designed for in-vitro studies, which are conducted outside of living organisms. In-vitro studies, derived from the Latin term "in glass," involve experiments performed in controlled laboratory settings using cells or tissues. It is important to note that these products are not categorized as medicines or drugs, and they have not received approval from the FDA for the prevention, treatment, or cure of any medical condition, ailment, or disease. We must emphasize that any form of bodily introduction of these products into humans or animals is strictly prohibited by law. It is essential to adhere to these guidelines to ensure compliance with legal and ethical standards in research and experimentation.