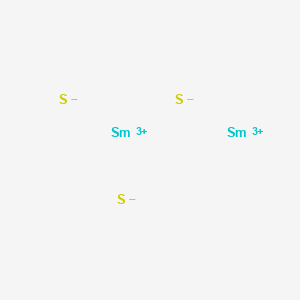
Samarium sulfide
Overview
Description
Samarium sulfide is a chemical compound composed of samarium and sulfur. It is represented by the chemical formula SmS. This compound is known for its unique properties, including a pressure-induced phase transition from a semiconducting to a metallic state.
Preparation Methods
Synthetic Routes and Reaction Conditions: Samarium sulfide can be synthesized using several methods. One common method involves the reaction of samarium metal with sulfur in an evacuated tube to prevent the loss of sulfur due to its low boiling point. The reaction is typically carried out at temperatures around 1000 K . Another method involves the reaction of samarium (II) bis[bis(trimethylsilyl)amide] with hydrogen sulfide in tetrahydrofuran at low pressure .
Industrial Production Methods: In industrial settings, this compound is often produced using high-temperature solid-state reactions. The reactants, samarium metal and sulfur, are mixed in stoichiometric proportions and heated in a furnace. The reaction is conducted in an inert atmosphere to prevent oxidation .
Chemical Reactions Analysis
Types of Reactions: Samarium sulfide undergoes various chemical reactions, including oxidation, reduction, and substitution reactions.
Common Reagents and Conditions:
Oxidation: this compound can be oxidized to form samarium oxide (Sm₂O₃) when exposed to air.
Reduction: It can be reduced back to samarium metal and sulfur under specific conditions.
Substitution: this compound can react with halogens to form samarium halides.
Major Products:
Oxidation: Samarium oxide (Sm₂O₃)
Reduction: Samarium metal and sulfur
Substitution: Samarium halides (e.g., SmCl₃, SmBr₃)
Scientific Research Applications
Samarium sulfide has several scientific research applications due to its unique properties:
Chemistry: It is used as a catalyst in various organic reactions, including the dehydration of alcohols.
Biology and Medicine: Samarium compounds, including this compound, are used in cancer treatments and as neutron absorbers in nuclear reactors.
Industry: this compound is used in high-density optical storage, memory materials, thermovoltaic devices, and infrared sensors
Mechanism of Action
Samarium sulfide can be compared with other lanthanide sulfides, such as europium sulfide (EuS) and gadolinium sulfide (GdS). While all these compounds exhibit interesting magnetic and electronic properties, this compound is unique due to its reversible pressure-induced phase transition. This property makes it particularly useful in applications requiring switchable materials .
Comparison with Similar Compounds
- Europium sulfide (EuS)
- Gadolinium sulfide (GdS)
- Samarium oxide (Sm₂O₃)
- Samarium halides (e.g., SmCl₃, SmBr₃)
Properties
IUPAC Name |
samarium(3+);trisulfide | |
---|---|---|
Source | PubChem | |
URL | https://pubchem.ncbi.nlm.nih.gov | |
Description | Data deposited in or computed by PubChem | |
InChI |
InChI=1S/3S.2Sm/q3*-2;2*+3 | |
Source | PubChem | |
URL | https://pubchem.ncbi.nlm.nih.gov | |
Description | Data deposited in or computed by PubChem | |
InChI Key |
KKZKWPQFAZAUSB-UHFFFAOYSA-N | |
Source | PubChem | |
URL | https://pubchem.ncbi.nlm.nih.gov | |
Description | Data deposited in or computed by PubChem | |
Canonical SMILES |
[S-2].[S-2].[S-2].[Sm+3].[Sm+3] | |
Source | PubChem | |
URL | https://pubchem.ncbi.nlm.nih.gov | |
Description | Data deposited in or computed by PubChem | |
Molecular Formula |
Sm2S3, S3Sm2 | |
Record name | Samarium(III) sulfide | |
Source | Wikipedia | |
URL | https://en.wikipedia.org/wiki/Samarium(III)_sulfide | |
Description | Chemical information link to Wikipedia. | |
Source | PubChem | |
URL | https://pubchem.ncbi.nlm.nih.gov | |
Description | Data deposited in or computed by PubChem | |
DSSTOX Substance ID |
DTXSID90923512 | |
Record name | Disamarium trisulphide | |
Source | EPA DSSTox | |
URL | https://comptox.epa.gov/dashboard/DTXSID90923512 | |
Description | DSSTox provides a high quality public chemistry resource for supporting improved predictive toxicology. | |
Molecular Weight |
396.9 g/mol | |
Source | PubChem | |
URL | https://pubchem.ncbi.nlm.nih.gov | |
Description | Data deposited in or computed by PubChem | |
CAS No. |
12067-22-0 | |
Record name | Samarium sulfide (Sm2S3) | |
Source | ChemIDplus | |
URL | https://pubchem.ncbi.nlm.nih.gov/substance/?source=chemidplus&sourceid=0012067220 | |
Description | ChemIDplus is a free, web search system that provides access to the structure and nomenclature authority files used for the identification of chemical substances cited in National Library of Medicine (NLM) databases, including the TOXNET system. | |
Record name | Samarium sulfide (Sm2S3) | |
Source | EPA Chemicals under the TSCA | |
URL | https://www.epa.gov/chemicals-under-tsca | |
Description | EPA Chemicals under the Toxic Substances Control Act (TSCA) collection contains information on chemicals and their regulations under TSCA, including non-confidential content from the TSCA Chemical Substance Inventory and Chemical Data Reporting. | |
Record name | Disamarium trisulphide | |
Source | EPA DSSTox | |
URL | https://comptox.epa.gov/dashboard/DTXSID90923512 | |
Description | DSSTox provides a high quality public chemistry resource for supporting improved predictive toxicology. | |
Record name | Disamarium trisulphide | |
Source | European Chemicals Agency (ECHA) | |
URL | https://echa.europa.eu/substance-information/-/substanceinfo/100.031.875 | |
Description | The European Chemicals Agency (ECHA) is an agency of the European Union which is the driving force among regulatory authorities in implementing the EU's groundbreaking chemicals legislation for the benefit of human health and the environment as well as for innovation and competitiveness. | |
Explanation | Use of the information, documents and data from the ECHA website is subject to the terms and conditions of this Legal Notice, and subject to other binding limitations provided for under applicable law, the information, documents and data made available on the ECHA website may be reproduced, distributed and/or used, totally or in part, for non-commercial purposes provided that ECHA is acknowledged as the source: "Source: European Chemicals Agency, http://echa.europa.eu/". Such acknowledgement must be included in each copy of the material. ECHA permits and encourages organisations and individuals to create links to the ECHA website under the following cumulative conditions: Links can only be made to webpages that provide a link to the Legal Notice page. | |
Disclaimer and Information on In-Vitro Research Products
Please be aware that all articles and product information presented on BenchChem are intended solely for informational purposes. The products available for purchase on BenchChem are specifically designed for in-vitro studies, which are conducted outside of living organisms. In-vitro studies, derived from the Latin term "in glass," involve experiments performed in controlled laboratory settings using cells or tissues. It is important to note that these products are not categorized as medicines or drugs, and they have not received approval from the FDA for the prevention, treatment, or cure of any medical condition, ailment, or disease. We must emphasize that any form of bodily introduction of these products into humans or animals is strictly prohibited by law. It is essential to adhere to these guidelines to ensure compliance with legal and ethical standards in research and experimentation.