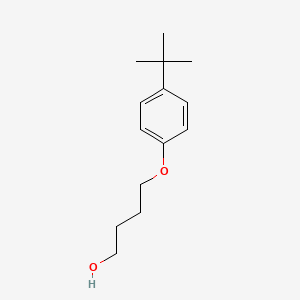
4-(4-Tert-butylphenoxy)butan-1-OL
- Click on QUICK INQUIRY to receive a quote from our team of experts.
- With the quality product at a COMPETITIVE price, you can focus more on your research.
Overview
Description
4-(4-Tert-butylphenoxy)butan-1-OL is a substituted butanol derivative featuring a tert-butylphenoxy group attached to the terminal carbon of a four-carbon alcohol chain.
Q & A
Q. What are the recommended synthetic pathways for 4-(4-tert-butylphenoxy)butan-1-ol in laboratory settings?
Basic Research Question
A common approach involves nucleophilic substitution between 4-tert-butylphenol and 1,4-dibromobutane under alkaline conditions. The reaction typically proceeds via an SN2 mechanism, where the phenoxide ion attacks the terminal carbon of the dibromobutane. Purification is achieved via column chromatography using silica gel and a hexane/ethyl acetate gradient. Yield optimization requires strict control of reaction temperature (60–80°C) and stoichiometric excess of 4-tert-butylphenol to minimize di-substitution byproducts .
Advanced Consideration : For enantioselective synthesis, chiral catalysts (e.g., BINOL-derived phosphoric acids) can induce asymmetry during key steps, though steric hindrance from the tert-butyl group may reduce efficiency. Kinetic studies using GC-MS or HPLC are critical to monitor intermediate formation .
Q. Which analytical techniques are most effective for structural elucidation and purity assessment of this compound?
Basic Research Question
- Nuclear Magnetic Resonance (NMR) : ¹H NMR (400 MHz, CDCl₃) reveals distinct signals for the tert-butyl group (δ 1.34 ppm, singlet) and the hydroxyl proton (δ 2.10 ppm, broad). ¹³C NMR confirms the quaternary carbon of the tert-butyl group (δ 31.2 ppm) .
- Gas Chromatography-Mass Spectrometry (GC-MS) : Retention time and fragmentation patterns (e.g., m/z 207 [M–H₂O]⁺) verify molecular identity .
- Infrared Spectroscopy (FTIR) : O–H stretch (3200–3600 cm⁻¹) and C–O–C ether linkage (1250 cm⁻¹) are diagnostic .
Advanced Consideration : High-resolution mass spectrometry (HRMS) and 2D NMR (e.g., COSY, HSQC) resolve ambiguities in complex mixtures, particularly when characterizing byproducts from incomplete substitution .
Q. How does the tert-butyl substituent influence the physical and chemical properties of this compound?
Basic Research Question
The bulky tert-butyl group enhances hydrophobicity (logP ≈ 3.2) compared to unsubstituted phenoxybutanols. This increases solubility in nonpolar solvents (e.g., hexane) but reduces aqueous solubility. Boiling point elevation (predicted ~280°C) is attributed to increased molecular weight and reduced volatility. Hydrogen bonding from the hydroxyl group moderates melting point (~45–50°C) .
Advanced Consideration : Computational studies (e.g., DFT) reveal steric effects that hinder rotational freedom in the phenoxy moiety, potentially altering reaction kinetics in oxidation or esterification pathways .
Q. What kinetic models explain the oxidation behavior of this compound under acidic conditions?
Advanced Research Question
In acidic Cr(VI)-mediated oxidation, the reaction follows pseudo-first-order kinetics. The rate constant (kobs) depends linearly on [Cr(VI)] and [substrate], with an Arrhenius activation energy (Ea) of ~65 kJ/mol. Steric hindrance from the tert-butyl group slows oxidation compared to linear-chain analogs. Competitive pathways (e.g., over-oxidation to carboxylic acids) are mitigated by controlling temperature (25–40°C) and Cr(VI) concentration .
Methodological Note : UV-Vis spectroscopy at 350 nm tracks Cr(VI) reduction, while GC-MS identifies intermediates like 4-(4-tert-butylphenoxy)butanal .
Q. How can researchers address discrepancies in reported biological activities of this compound?
Advanced Research Question
Contradictions in enzyme inhibition studies (e.g., IC₅₀ variability) often arise from differences in assay conditions (pH, co-solvents) or impurity profiles. Strategies include:
Purity Validation : Use HPLC with diode-array detection (DAD) to ensure >98% purity .
Solvent Optimization : Replace DMSO with ethanol to avoid solvent-enzyme interactions .
Dose-Response Curves : Test across a wider concentration range (0.1–100 µM) to account for non-linear effects.
Case Study : In cytochrome P450 inhibition assays, discrepancies were resolved by standardizing incubation times and pre-equilibrating substrates .
Q. What mechanistic insights exist for the interaction of this compound with lipid bilayers or protein targets?
Advanced Research Question
Molecular dynamics simulations suggest that the tert-butyl group anchors the compound to lipid bilayer hydrophobic cores, while the hydroxyl group forms hydrogen bonds with phospholipid headgroups. This dual interaction modulates membrane fluidity, as shown by fluorescence anisotropy assays using DPH probes. In protein binding, NMR titration experiments reveal preferential interaction with hydrophobic pockets (e.g., serum albumin), with binding constants (Kd) in the µM range .
Comparison with Similar Compounds
Structural and Functional Comparison with Similar Compounds
Substituted Butanols with Aromatic Moieties
4-(4-Methylphenyl)butan-1-OL ()
- Structure: A butanol chain linked to a 4-methylphenyl group.
- Key Differences : Lacks the tert-butyl and ether oxygen present in the target compound.
- Applications : Used in research and production due to its aromatic hydrophobicity .
4-([1,1'-Biphenyl]-4-yl)butan-1-OL ()
- Structure: A biphenyl group attached to butanol.
4-(n-Heptyloxy)butan-1-OL ()
- Structure: A linear heptyl ether chain attached to butanol.
- Key Differences: The long alkoxy chain enhances flexibility and volatility compared to the rigid tert-butylphenoxy group. Used as a pheromone component in beetles .
Halogenated and Functionalized Butanols
4-Fluoro-1-butanol ()
- Structure: A fluorine atom at the terminal carbon of butanol.
- Key Differences: Smaller substituent (fluorine vs. tert-butylphenoxy) results in lower molecular weight (94.11 g/mol) and higher polarity. Exhibits irritant properties, requiring stringent safety protocols .
4-(tert-Butyldimethylsilanyloxy)butan-1-OL ()
- Structure: A silyl-protected butanol derivative.
- Key Differences : The tert-butyldimethylsilyl (TBS) group serves as a protecting group in synthesis, contrasting with the target compound’s stable ether linkage. NMR data (δ 0.90 ppm for tert-butyl protons) suggests similarities in shielding effects .
Physicochemical Properties
Compound | Molecular Formula | Molecular Weight (g/mol) | Key Features |
---|---|---|---|
4-(4-Tert-butylphenoxy)butan-1-OL* | C₁₄H₂₂O₂ | ~234.3 (estimated) | High lipophilicity, rigid aromatic ether |
4-(4-Methylphenyl)butan-1-OL | C₁₁H₁₆O | 164.24 | Moderate hydrophobicity, simple aryl group |
4-Fluoro-1-butanol | C₄H₉FO | 94.11 | Polar, volatile, irritant |
4-(n-Heptyloxy)butan-1-OL | C₁₁H₂₄O₂ | 200.31 | Flexible alkoxy chain, pheromone activity |
*Estimated based on structural analogs.
Analytical Characterization
Preparation Methods
Williamson Ether Synthesis
The Williamson ether synthesis is a classical method for ether formation, involving the reaction of an alkoxide ion with an alkyl halide or sulfonate. For 4-(4-tert-butylphenoxy)butan-1-OL, this approach requires 4-tert-butylphenol and 4-halobutanol (e.g., 4-bromo-1-butanol) as precursors.
Reaction Mechanism
-
Deprotonation : 4-tert-butylphenol is treated with a strong base (e.g., NaOH or KOH) to generate the phenoxide ion.
-
Nucleophilic Substitution : The phenoxide attacks the electrophilic carbon of 4-bromo-1-butanol, displacing the bromide ion.
Optimization Considerations
-
Leaving Group Efficiency : Bromides or tosylates are preferred due to their superior leaving-group ability compared to chlorides .
-
Solvent Choice : Polar aprotic solvents like dimethylformamide (DMF) enhance reaction rates by stabilizing ionic intermediates .
-
Temperature : Mild heating (40–60°C) mitigates side reactions such as elimination, which is prevalent in β-hydroxy alkyl halides .
Limitations
-
Instability of 4-Bromo-1-butanol : The proximity of the hydroxyl and halogen groups in 4-bromo-1-butanol increases the risk of intramolecular elimination, forming butene derivatives. Tosylate derivatives (e.g., 4-tosyloxy-1-butanol) offer improved stability .
Mitsunobu Reaction
The Mitsunobu reaction enables ether formation under mild, non-basic conditions, utilizing a diazo compound (e.g., diethyl azodicarboxylate, DEAD) and triphenylphosphine (Ph₃P). This method is advantageous for coupling sterically hindered phenols like 4-tert-butylphenol with diols.
Procedure
-
Diol Protection : One hydroxyl group of 1,4-butanediol is protected (e.g., as a tert-butyldimethylsilyl ether) to ensure regioselectivity.
-
Coupling : The free hydroxyl reacts with 4-tert-butylphenol in the presence of DEAD and Ph₃P.
-
Deprotection : The silyl ether is cleaved using tetrabutylammonium fluoride (TBAF).
Advantages
-
Regioselectivity : Protection-deprotection strategies prevent undesired side reactions .
-
Yield : Mitsunobu reactions typically achieve >80% yields for sterically accessible substrates .
Reduction of Ester Intermediates
This two-step method involves synthesizing an ester intermediate followed by reduction to the alcohol.
Step 1: Esterification
4-tert-Butylphenol reacts with ethyl 4-bromobutanoate in the presence of a base (e.g., K₂CO₃) to form ethyl 4-(4-tert-butylphenoxy)butanoate . Zinc chloride (ZnCl₂) catalysis, as demonstrated in the synthesis of 4-chlorobutanol esters , may enhance reaction efficiency.
Step 2: Reduction
The ester is reduced to the primary alcohol using lithium aluminum hydride (LiAlH₄). For example, LiAlH₄ reduction of 4,4-bis(4-fluorophenyl)butanoic acid achieved a 92.6% yield .
Challenges
-
Over-reduction : Excess LiAlH₄ may reduce aromatic rings, necessitating strict stoichiometric control .
Acid-Catalyzed Etherification
Solid acid catalysts, such as acid-activated montmorillonite, facilitate direct etherification between 4-tert-butylphenol and 1,4-butanediol. This method mirrors the alkylation of phenol with 4-hydroxybutan-2-one reported in US Patent 8,471,068 .
Reaction Conditions
-
Catalyst : Acid-treated montmorillonite (surface acidity: 0.4–0.6 mmol/g) provides high selectivity (75–81%) for ether products .
-
Solvent : Solvent-free conditions or 2-methyltetrahydrofuran improve mass transfer .
Drawbacks
-
Regioselectivity : Competing reactions at both hydroxyls of 1,4-butanediol necessitate excess diol or selective protection .
Comparative Analysis of Methods
Method | Catalyst/Reagents | Yield (%) | Key Advantage | Limitation |
---|---|---|---|---|
Williamson Synthesis | NaOH, 4-bromo-1-butanol | 70–85 | Simplicity | Elimination side reactions |
Mitsunobu Reaction | DEAD, Ph₃P | 80–90 | High regioselectivity | Costly reagents |
Ester Reduction | ZnCl₂, LiAlH₄ | 65–75 | Scalable | Multi-step synthesis |
Acid-Catalyzed | Montmorillonite | 60–75 | Solvent-free conditions | Moderate selectivity |
Experimental Optimization Strategies
Minimizing Elimination in Williamson Synthesis
-
Low-Temperature Reactions : Conducting the reaction at 0–10°C reduces elimination, as seen in the synthesis of 4-chlorobutanol esters .
-
Phase-Transfer Catalysis : Tetrabutylammonium bromide (TBAB) enhances interfacial contact between aqueous and organic phases .
Enhancing Catalyst Efficiency in Acid-Catalyzed Methods
Properties
CAS No. |
64673-24-1 |
---|---|
Molecular Formula |
C14H22O2 |
Molecular Weight |
222.32 g/mol |
IUPAC Name |
4-(4-tert-butylphenoxy)butan-1-ol |
InChI |
InChI=1S/C14H22O2/c1-14(2,3)12-6-8-13(9-7-12)16-11-5-4-10-15/h6-9,15H,4-5,10-11H2,1-3H3 |
InChI Key |
JZGBOFLIJVDYMR-UHFFFAOYSA-N |
Canonical SMILES |
CC(C)(C)C1=CC=C(C=C1)OCCCCO |
Origin of Product |
United States |
Disclaimer and Information on In-Vitro Research Products
Please be aware that all articles and product information presented on BenchChem are intended solely for informational purposes. The products available for purchase on BenchChem are specifically designed for in-vitro studies, which are conducted outside of living organisms. In-vitro studies, derived from the Latin term "in glass," involve experiments performed in controlled laboratory settings using cells or tissues. It is important to note that these products are not categorized as medicines or drugs, and they have not received approval from the FDA for the prevention, treatment, or cure of any medical condition, ailment, or disease. We must emphasize that any form of bodily introduction of these products into humans or animals is strictly prohibited by law. It is essential to adhere to these guidelines to ensure compliance with legal and ethical standards in research and experimentation.