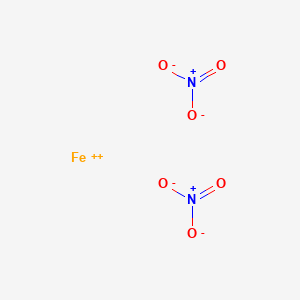
Iron nitrate
Overview
Description
Iron nitrate, known by its chemical formula as Fe(NO₃)₂, is a significant inorganic compound frequently utilized in various scientific and industrial processes. It is an inorganic salt derived from the reaction of nitric acid and iron metal, typically manifesting as a light green or pale violet solid . This compound is one of the two commonly available nitrates of iron, the other being iron(III) nitrate .
Synthetic Routes and Reaction Conditions:
- The most common method to prepare iron(II) nitrate involves the reaction of iron with dilute nitric acid, resulting in iron(II) nitrate and the release of hydrogen gas .
- Another prevalent method involves dissolving iron(II) sulfate in nitric acid, which then gives iron(II) nitrate and sulfuric acid .
Industrial Production Methods:
- Industrially, iron(II) nitrate can be produced by reacting iron metal with cold dilute nitric acid. This reaction can produce different hydrates depending on the temperature conditions .
Types of Reactions:
Oxidation: this compound can undergo oxidation to form iron(III) nitrate.
Reduction: It can be reduced to iron metal under specific conditions.
Substitution: this compound can participate in substitution reactions where the nitrate ions are replaced by other anions.
Common Reagents and Conditions:
Oxidation: Typically involves oxidizing agents like oxygen or other oxidizing compounds.
Reduction: Often requires reducing agents such as hydrogen gas or other reducing agents.
Substitution: Involves reagents that can provide the substituting anions.
Major Products:
Oxidation: Iron(III) nitrate.
Reduction: Iron metal.
Substitution: Various iron salts depending on the substituting anion.
Scientific Research Applications
Agricultural Applications
Biofortification
Iron nitrate is widely used to enhance the nutritional quality of crops through biofortification. A study on Swiss chard (Beta vulgaris L.) demonstrated that foliar applications of this compound significantly increased the iron content in the plant tissues. The research indicated that higher concentrations (5000 mg L^-1) resulted in a 226% increase in iron accumulation compared to control treatments, while also affecting protein and nitrate levels in the plants .
Table 1: Effects of this compound on Nutrient Accumulation in Swiss Chard
Treatment Concentration (mg L^-1) | Iron Accumulation (%) | Protein Accumulation (%) | Nitrate Accumulation (%) |
---|---|---|---|
Control (0) | 0 | 0 | 0 |
2500 | 120 | 80 | 50 |
5000 | 226 | 70 | 100 |
Soil Health Improvement
this compound also plays a critical role in improving soil health by facilitating the availability of iron, which is essential for plant growth. Its application can enhance the microbial activity in the soil, promoting better nutrient cycling and plant uptake .
Catalytic Applications
Fischer-Tropsch Synthesis
this compound is utilized as a precursor for synthesizing iron catalysts used in Fischer-Tropsch synthesis, which converts syngas into hydrocarbons. Research indicates that this compound can be combined with nickel nitrate to optimize catalyst performance, enhancing the production of light olefins .
Table 2: Comparison of Catalyst Performance Using Different Precursors
Precursor Type | Light Olefin Yield (%) | Catalyst Activity (g CO/h/g catalyst) |
---|---|---|
This compound + Nickel | 45 | 20 |
Iron Oxide | 40 | 18 |
Nickel Nitrate | 35 | 15 |
Environmental Applications
Remediation of Contaminated Soils
this compound has been investigated for its potential in remediating contaminated soils, particularly those affected by heavy metals. The compound aids in the reduction of metal ions, facilitating their immobilization and thereby reducing their bioavailability .
Microbial Redox Cycling
In environmental microbiology, this compound is involved in microbial redox cycling processes. Studies have shown that it can serve as an electron acceptor in anaerobic conditions, promoting the biogeochemical cycling of nutrients like nitrogen and phosphorus .
Case Studies
Case Study: this compound in Wheat and Pea Intercropping
A recent study evaluated the use of nano iron and this compound in intercropping systems involving wheat and peas. The findings revealed that nano iron applications resulted in higher fertilizer use efficiency compared to conventional this compound applications, indicating a shift towards more sustainable agricultural practices .
Mechanism of Action
Iron nitrate exerts its effects primarily through its ability to act as an oxidizing agent. The iron ion (Fe²⁺) can participate in redox reactions, where it can either donate or accept electrons, thus facilitating various chemical transformations. The nitrate ions (NO₃⁻) can also participate in reactions, contributing to the overall reactivity of the compound .
Comparison with Similar Compounds
Iron(III) nitrate (Fe(NO₃)₃): Another commonly available nitrate of iron, which is more stable and commercially available compared to iron(II) nitrate.
Copper(II) nitrate (Cu(NO₃)₂): Similar in its nitrate composition but involves copper instead of iron.
Zinc nitrate (Zn(NO₃)₂): Another nitrate compound involving zinc.
Uniqueness:
- Iron nitrate is unique due to its specific oxidation state of iron (Fe²⁺), which gives it distinct chemical properties and reactivity compared to iron(III) nitrate (Fe³⁺). Its ability to act as a reducing agent in certain conditions also sets it apart from other nitrate compounds .
Biological Activity
Iron nitrate, particularly in its ferric form (Fe(NO₃)₃), plays a significant role in various biological processes, especially in microbial metabolism and environmental biogeochemistry. This article explores the biological activity of this compound, emphasizing its involvement in nitrate reduction, microbial growth enhancement, and potential applications in bioremediation.
Ferric nitrate appears as a violet crystalline solid and is known for its non-combustible nature while being capable of accelerating the burning of combustible materials . Its solubility in water makes it a suitable candidate for various biological applications.
Role in Nitrate Reduction
This compound serves as an essential component in the microbial reduction of nitrates. This process is crucial for nitrogen cycling in ecosystems, particularly in anaerobic conditions where microorganisms utilize nitrates as electron acceptors.
- Microbial Mediation : Certain bacteria, such as Shewanella oneidensis, can reduce nitrate to nitrite and further to nitrogen gas using iron as a co-factor. This process not only facilitates nitrogen removal from environments but also enhances the bioavailability of iron .
- Enzymatic Activity : The presence of iron significantly increases the activity of nitrate and nitrite reductases. For instance, studies have shown that increasing iron concentrations can enhance the activity of these enzymes by several folds .
Study 1: Nitrate Reduction by Shewanella oneidensis
In a controlled experiment, S. oneidensis was incubated with varying concentrations of nitrate and lactate. The results indicated that 90% of nitrate was reduced within 12 hours at initial concentrations of 30 mg·L⁻¹ NO₃⁻-N . The study highlighted the importance of biogenic Fe(II) in promoting this reduction process.
Study 2: Iron Enhancement on Nitrate Utilization
A study investigated the effects of added ferric ions on the activities of nitrate and nitrite reductases. The results demonstrated that higher concentrations of Fe³⁺ significantly accelerated both nitrate utilization and nitrite accumulation, showcasing the synergistic effect of iron on microbial metabolic pathways .
Research Findings
Research has consistently shown that iron is a critical trace element that influences microbial growth and activity related to nitrogen metabolism.
Table 1: Effect of Iron Concentration on Reductase Activity
Fe Amendment (μM) | Nitrate Reductase Activity (nmol min⁻¹ mg⁻¹) | Nitrite Reductase Activity (nmol min⁻¹ mg⁻¹) |
---|---|---|
0 | 19 | 90 |
50 | 63 | 100 |
250 | 89 | 2900 |
500 | 89 | 6100 |
This table illustrates how increasing iron concentrations correlate with enhanced enzymatic activity, emphasizing the vital role iron plays in microbial metabolism .
Implications for Bioremediation
The biological activity associated with this compound has significant implications for environmental remediation strategies. The ability to enhance nitrate reduction through iron supplementation can be harnessed for treating contaminated water bodies, thereby reducing excess nitrates that contribute to eutrophication.
Properties
IUPAC Name |
iron(2+);dinitrate | |
---|---|---|
Source | PubChem | |
URL | https://pubchem.ncbi.nlm.nih.gov | |
Description | Data deposited in or computed by PubChem | |
InChI |
InChI=1S/Fe.2NO3/c;2*2-1(3)4/q+2;2*-1 | |
Source | PubChem | |
URL | https://pubchem.ncbi.nlm.nih.gov | |
Description | Data deposited in or computed by PubChem | |
InChI Key |
MVFCKEFYUDZOCX-UHFFFAOYSA-N | |
Source | PubChem | |
URL | https://pubchem.ncbi.nlm.nih.gov | |
Description | Data deposited in or computed by PubChem | |
Canonical SMILES |
[N+](=O)([O-])[O-].[N+](=O)([O-])[O-].[Fe+2] | |
Source | PubChem | |
URL | https://pubchem.ncbi.nlm.nih.gov | |
Description | Data deposited in or computed by PubChem | |
Molecular Formula |
FeN2O6 | |
Source | PubChem | |
URL | https://pubchem.ncbi.nlm.nih.gov | |
Description | Data deposited in or computed by PubChem | |
DSSTOX Substance ID |
DTXSID70431140 | |
Record name | Ferrous nitrate | |
Source | EPA DSSTox | |
URL | https://comptox.epa.gov/dashboard/DTXSID70431140 | |
Description | DSSTox provides a high quality public chemistry resource for supporting improved predictive toxicology. | |
Molecular Weight |
179.86 g/mol | |
Source | PubChem | |
URL | https://pubchem.ncbi.nlm.nih.gov | |
Description | Data deposited in or computed by PubChem | |
CAS No. |
14013-86-6 | |
Record name | Ferrous nitrate | |
Source | EPA DSSTox | |
URL | https://comptox.epa.gov/dashboard/DTXSID70431140 | |
Description | DSSTox provides a high quality public chemistry resource for supporting improved predictive toxicology. | |
Synthesis routes and methods
Procedure details
Disclaimer and Information on In-Vitro Research Products
Please be aware that all articles and product information presented on BenchChem are intended solely for informational purposes. The products available for purchase on BenchChem are specifically designed for in-vitro studies, which are conducted outside of living organisms. In-vitro studies, derived from the Latin term "in glass," involve experiments performed in controlled laboratory settings using cells or tissues. It is important to note that these products are not categorized as medicines or drugs, and they have not received approval from the FDA for the prevention, treatment, or cure of any medical condition, ailment, or disease. We must emphasize that any form of bodily introduction of these products into humans or animals is strictly prohibited by law. It is essential to adhere to these guidelines to ensure compliance with legal and ethical standards in research and experimentation.