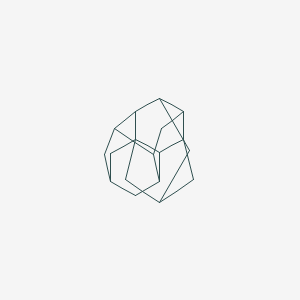
Triamantane
- Click on QUICK INQUIRY to receive a quote from our team of experts.
- With the quality product at a COMPETITIVE price, you can focus more on your research.
Overview
Description
Triamantane (C₁₈H₂₄) is a three-cage diamondoid, a hydrocarbon with a carbon skeleton that mimics the tetrahedral lattice of diamond. Diamondoids are defined by their strain-free, thermally stable structures, with this compound occupying a critical position between lower (adamantane, diamantane) and higher (tetramantane, pentamantane) homologs. Its melting point (221.5°C) is lower than adamantane (269°C) and diamantane (236.5°C), reflecting subtle thermodynamic variations with cage addition . This compound’s unique electronic, thermal, and structural properties make it a pivotal compound for studying nanoscale diamond-like materials and their applications in nanotechnology, geochemistry, and biomedicine.
Scientific Research Applications
Diamond Synthesis
Facile Diamond Formation
Triamantane has been identified as a highly effective precursor for diamond synthesis. Research indicates that when subjected to high pressure and temperature conditions, this compound converts to diamond more readily than other diamondoids such as adamantane and diamantane. Specifically, it requires the lowest pressure-temperature (P-T) conditions for diamond formation, demonstrating a significant reduction in both energy and time barriers for this transformation .
Experimental Findings
In experiments using laser-induced high-pressure techniques, this compound was shown to transform into diamond at around 20 GPa and 2000 K. The resulting diamonds exhibited well-faceted euhedral crystal forms, indicating a successful transition from the diamondoid structure to crystalline diamond . The transformation kinetics were also favorable; this compound displayed the fastest transition time among the lower diamondoids studied .
Nanotechnology Applications
Nanomaterials Development
this compound's unique structural characteristics lend themselves to applications in nanotechnology. Its ability to form stable nanostructures can be utilized in creating advanced materials with specific mechanical and thermal properties. The synthesis of diamond from this compound opens pathways for producing high-performance nanomaterials that can be used in various applications, including electronics and coatings .
Carbon-Based Nanomaterials
The conversion of this compound into diamond nanostructures allows for the development of carbon-based nanomaterials that can exhibit superior hardness and thermal conductivity compared to conventional materials. This property is particularly valuable in industries requiring durable materials, such as aerospace and automotive sectors .
Biomedical Applications
Drug Delivery Systems
The stability and biocompatibility of diamondoids like this compound make them suitable candidates for drug delivery systems. Their cage-like structure can encapsulate therapeutic agents, allowing for controlled release and targeted delivery within biological systems. This application is particularly promising in cancer therapy, where targeted drug delivery is crucial for minimizing side effects while maximizing therapeutic efficacy .
Antiviral Activity
Research has also indicated potential pharmacological activities associated with derivatives of this compound. For example, compounds derived from this compound have shown antiviral properties against certain viruses, suggesting possible applications in developing antiviral medications .
Material Science
Structural Applications
Due to its robust structure, this compound can serve as a building block for synthesizing new materials with enhanced properties. Its ability to form stable frameworks can lead to innovations in material science, particularly in creating composites that require high strength-to-weight ratios or exceptional thermal stability .
Summary Table of this compound Applications
Application Area | Description | Key Findings |
---|---|---|
Diamond Synthesis | Converts to diamond under high P-T conditions with low energy barriers | Fastest transition time among lower diamondoids; forms euhedral diamonds |
Nanotechnology | Forms stable nanostructures useful in advanced materials | Potential for high-performance carbon-based nanomaterials |
Biomedical Applications | Suitable for drug delivery systems; potential antiviral properties | Encapsulation capabilities; promising results against certain viruses |
Material Science | Acts as a building block for new materials with enhanced properties | Innovations in composites with high strength-to-weight ratios |
Q & A
Basic Research Questions
Q. What established synthetic methodologies are used to produce high-purity Triamantane, and how do experimental parameters (e.g., temperature, catalysts) influence yield?
- Methodological Answer : this compound synthesis typically involves chemical vapor deposition (CVD) or solution-phase methods. For CVD, parameters like substrate temperature (optimized at 400–600°C) and hydrocarbon precursors (e.g., adamantane derivatives) directly affect crystallinity and yield . Solution-phase synthesis often employs halogenated precursors with reducing agents (e.g., LiAlH₄), where reaction time and stoichiometry must be tightly controlled to minimize byproducts. Purity is assessed via gas chromatography-mass spectrometry (GC-MS) and high-performance liquid chromatography (HPLC), with yields ranging from 15–35% depending on conditions .
Q. Which spectroscopic and crystallographic techniques are prioritized for validating this compound’s molecular structure?
- Methodological Answer : Nuclear magnetic resonance (¹³C NMR) and infrared (IR) spectroscopy are critical for identifying functional groups and bonding patterns. For crystallographic validation, single-crystal X-ray diffraction (SCXRD) is the gold standard, though powder XRD is used for bulk samples. Differential scanning calorimetry (DSC) complements these methods by analyzing thermal stability, with melting points typically reported between 250–300°C .
Advanced Research Questions
Q. How can computational models (e.g., DFT, molecular dynamics) reconcile discrepancies in this compound’s reported thermodynamic properties (e.g., enthalpy of formation)?
- Methodological Answer : Density functional theory (DFT) simulations at the B3LYP/6-311G(d,p) level can calculate formation enthalpies, but discrepancies arise from basis set choices and solvation models. Researchers should cross-validate computational results with experimental calorimetry data, ensuring solvent effects and lattice energies are accounted for. For example, DFT-predicted enthalpies may deviate by ±5 kJ/mol from experimental values, requiring iterative refinement of computational parameters .
Q. What systematic approaches address contradictions in the catalytic activity of this compound-based nanomaterials?
- Methodological Answer : Contradictions often stem from variations in surface functionalization or defect density. A robust protocol includes:
- Synthesis Control : Adjusting CVD parameters to regulate defect sites (e.g., vacancies, dopants).
- In-Situ Characterization : Using Raman spectroscopy or X-ray photoelectron spectroscopy (XPS) to monitor surface chemistry during catalysis.
- Triangulation : Comparing catalytic performance across multiple substrates (e.g., Au vs. Pt supports) and reaction conditions (pH, temperature) to isolate variables .
Q. How can researchers design experiments to evaluate this compound’s electronic properties (e.g., bandgap) for nanoelectronic applications?
- Methodological Answer : Ultraviolet photoelectron spectroscopy (UPS) and scanning tunneling microscopy (STM) are key for measuring work function and band alignment. For bulk samples, spectroscopic ellipsometry provides optical bandgap data. To ensure reproducibility, experiments should include control samples (e.g., adamantane) and account for environmental factors (e.g., humidity-induced surface oxidation) .
Q. Data Reliability and Contradiction Management
Q. What validation frameworks are recommended to ensure consistency in this compound’s reported mechanical properties (e.g., Young’s modulus)?
- Methodological Answer : Triangulate nanoindentation results with atomic force microscopy (AFM) measurements and molecular dynamics simulations. For example, Young’s modulus values vary between 80–120 GPa due to anisotropic crystal orientations; researchers should specify crystallographic planes tested and use ≥3 independent samples per condition .
Q. How should conflicting data on this compound’s solubility in polar solvents be resolved?
- Methodological Answer : Employ a phased approach:
Replicate Experiments : Use standardized solvents (e.g., DMSO, ethanol) and purity thresholds (>99%).
Advanced Analytics : Pair gravimetric analysis with UV-Vis spectroscopy to quantify dissolved fractions.
Theoretical Modeling : Apply Hansen solubility parameters (HSPs) to predict solvent compatibility, then correlate with empirical data .
Q. Methodological Best Practices
- Data Tables : Include comparative tables synthesizing key findings (e.g., Table 1: Synthesis Methods for this compound: Yield vs. Purity).
- Transparency : Document all experimental parameters (e.g., CVD pressure, precursor ratios) to enable replication .
- Ethical Considerations : Adhere to FINER criteria (Feasible, Interesting, Novel, Ethical, Relevant) when framing questions .
Comparison with Similar Compounds
Comparison with Lower Diamondoids (Adamantane and Diamantane)
Structural and Thermal Properties
- Melting Points : Adamantane (C₁₀H₁₆) exhibits the highest melting point (269°C), followed by diamantane (C₁₄H₂₀, 236.5°C) and triamantane (221.5°C). This trend correlates with decreasing molecular symmetry and increased conformational flexibility as cages are added .
- Phase Transitions : this compound undergoes a crystalline phase transition (II→I) with ΔH = 1.06 kJ/mol and ΔS = 3.77 kJ/mol·K at 293.65 K. Comparable data for adamantane and diamantane are scarce, but this compound’s lower thermal stability suggests enhanced molecular mobility .
Electronic and Optical Properties
- Absorption Spectra : Adamantane and diamantane exhibit sharp, discrete peaks in their electronic spectra, characteristic of molecular-like excitations. This compound, however, shows a quasicontinuous band structure due to closer energy spacing of electronic states, marking the onset of bulk-like behavior .
- Excited-State Dynamics : Quantum Monte Carlo (QMC) simulations reveal negligible changes in C–H bond lengths between ground and excited states for tetramantane, but this compound already demonstrates reduced vibronic coupling compared to adamantane, facilitating smoother electronic transitions .
Biochemical Interactions
- Cytochrome P-450 Binding : this compound’s bulkier structure results in a higher dissociation constant (Ks = 4.3 µmol/L) compared to diamantane (Ks = 0.5 µmol/L) and adamantane (Ks = 1.3 µmol/L), indicating poorer fit within enzyme active sites .
Comparison with Higher Diamondoids (Tetramantane and Beyond)
Structural Complexity and Isomerism
- This escalating isomerism complicates synthesis and purification of higher diamondoids .
Electronic Properties
- Band-Like Absorption : Tetramantane and higher diamondoids exhibit fully quasicontinuous absorption spectra, whereas this compound represents the transitional point where discrete peaks coalesce into bands .
Thermal and Phase Transition Properties
Electronic and Optical Properties
- Stokes Shift : Density functional theory (DFT) and QMC calculations show this compound’s Stokes shift (490 meV) is smaller than adamantane’s (650 meV), indicating reduced structural relaxation upon photoexcitation .
- Charge Density Distribution : this compound’s electron-hole orbitals exhibit delocalization across multiple cages, a precursor to the bulk-like behavior seen in higher diamondoids .
Nanotechnology
- Diamond Synthesis : this compound reorganizes into diamond under high pressure and laser heating, requiring less energy than adamantane or diamantane due to its preorganized three-cage structure .
- Nanowires: Electrophoretic assembly of this compound forms vertically aligned nanowires, a precursor for hierarchical nanostructures in optoelectronics .
Biotechnology
- DNA Modification : this compound-modified nucleotides integrate into DNA without disrupting helical conformation, enabling stable biomolecular probes .
- Polymer Additives : this compound enhances thermal stability and rigidity in polypropylene and polycarbonate composites, outperforming adamantane in stress-strain resilience .
Geochemical Significance
This compound serves as a biomarker in petroleum analysis. Quantitative extended diamondoid analysis (QEDA) uses this compound as a reference to differentiate marine vs. terrigenous oil sources. For example, R-706 oil shows higher polymantane concentrations than TJ-1323, indicating distinct maturation pathways .
Data Tables
Table 1: Physical and Thermal Properties of Diamondoids
Compound | Molecular Formula | Melting Point (°C) | Phase Transition ΔH (kJ/mol) | Phase Transition ΔS (kJ/mol·K) |
---|---|---|---|---|
Adamantane | C₁₀H₁₆ | 269 | N/A | N/A |
Diamantane | C₁₄H₂₀ | 236.5 | N/A | N/A |
This compound | C₁₈H₂₄ | 221.5 | 1.06 | 3.77 |
Tetramantane | C₂₂H₂₈ | ~200 (estimated) | N/A | N/A |
Properties
CAS No. |
13349-10-5 |
---|---|
Molecular Formula |
C18H24 |
Molecular Weight |
240.4 g/mol |
IUPAC Name |
heptacyclo[7.7.1.13,15.01,12.02,7.04,13.06,11]octadecane |
InChI |
InChI=1S/C18H24/c1-8-2-13-10-5-11-14-3-9-4-15(11)17(13)18(6-8,7-9)16(14)12(1)10/h8-17H,1-7H2 |
InChI Key |
AMFOXYRZVYMNIR-UHFFFAOYSA-N |
SMILES |
C1C2CC3C4C1C5C6CC7CC(C6C4)C3C5(C2)C7 |
Canonical SMILES |
C1C2CC3C4C1C5C6CC7CC(C6C4)C3C5(C2)C7 |
Origin of Product |
United States |
Disclaimer and Information on In-Vitro Research Products
Please be aware that all articles and product information presented on BenchChem are intended solely for informational purposes. The products available for purchase on BenchChem are specifically designed for in-vitro studies, which are conducted outside of living organisms. In-vitro studies, derived from the Latin term "in glass," involve experiments performed in controlled laboratory settings using cells or tissues. It is important to note that these products are not categorized as medicines or drugs, and they have not received approval from the FDA for the prevention, treatment, or cure of any medical condition, ailment, or disease. We must emphasize that any form of bodily introduction of these products into humans or animals is strictly prohibited by law. It is essential to adhere to these guidelines to ensure compliance with legal and ethical standards in research and experimentation.