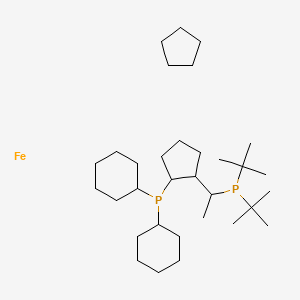
MFCD08543435
- Click on QUICK INQUIRY to receive a quote from our team of experts.
- With the quality product at a COMPETITIVE price, you can focus more on your research.
Overview
Description
®-1-[(S)-2-(Dicyclohexylphosphino)ferrocenyl]ethyli-tert-butylphosphine is a chiral phosphine ligand that is widely used in asymmetric catalysis. This compound is known for its ability to induce high enantioselectivity in various catalytic reactions, making it a valuable tool in the synthesis of chiral molecules.
Preparation Methods
Synthetic Routes and Reaction Conditions
The synthesis of ®-1-[(S)-2-(Dicyclohexylphosphino)ferrocenyl]ethyli-tert-butylphosphine typically involves the reaction of a ferrocene derivative with a phosphine reagent. One common method involves the reaction of ®-1-(ferrocenyl)ethanol with (S)-2-(dicyclohexylphosphino)ethyl chloride under basic conditions. The reaction is usually carried out in an inert atmosphere to prevent oxidation of the phosphine.
Industrial Production Methods
Industrial production of this compound often involves similar synthetic routes but on a larger scale. The use of continuous flow reactors and automated systems can enhance the efficiency and yield of the synthesis. Additionally, purification techniques such as recrystallization and chromatography are employed to obtain the compound in high purity.
Chemical Reactions Analysis
Types of Reactions
®-1-[(S)-2-(Dicyclohexylphosphino)ferrocenyl]ethyli-tert-butylphosphine undergoes various types of reactions, including:
Oxidation: The phosphine group can be oxidized to form phosphine oxides.
Substitution: The compound can participate in substitution reactions where the phosphine ligand is replaced by other ligands.
Coordination: The compound can coordinate with metal centers to form metal-phosphine complexes.
Common Reagents and Conditions
Common reagents used in reactions with this compound include oxidizing agents like hydrogen peroxide for oxidation reactions and various metal salts for coordination reactions. The reactions are typically carried out under inert conditions to prevent unwanted side reactions.
Major Products
The major products formed from these reactions include phosphine oxides, substituted phosphine derivatives, and metal-phosphine complexes, which are often used as catalysts in various chemical transformations.
Scientific Research Applications
®-1-[(S)-2-(Dicyclohexylphosphino)ferrocenyl]ethyli-tert-butylphosphine has a wide range of applications in scientific research:
Chemistry: It is used as a ligand in asymmetric catalysis to synthesize chiral molecules with high enantioselectivity.
Biology: The compound is used in the study of enzyme mechanisms and the development of enzyme inhibitors.
Medicine: It is employed in the synthesis of chiral drugs and pharmaceuticals.
Industry: The compound is used in the production of fine chemicals and agrochemicals.
Mechanism of Action
The mechanism by which ®-1-[(S)-2-(Dicyclohexylphosphino)ferrocenyl]ethyli-tert-butylphosphine exerts its effects involves the coordination of the phosphine ligand to a metal center. This coordination activates the metal center, allowing it to catalyze various chemical reactions. The chiral nature of the ligand induces enantioselectivity in the catalytic process, leading to the formation of chiral products.
Comparison with Similar Compounds
Similar Compounds
- ®-1-[(S)-2-(Diphenylphosphino)ferrocenyl]ethyli-tert-butylphosphine
- ®-1-[(S)-2-(Di-tert-butylphosphino)ferrocenyl]ethyli-tert-butylphosphine
- ®-1-[(S)-2-(Dicyclohexylphosphino)ferrocenyl]ethyli-methylphosphine
Uniqueness
®-1-[(S)-2-(Dicyclohexylphosphino)ferrocenyl]ethyli-tert-butylphosphine is unique due to its high steric bulk and strong electron-donating properties, which enhance its ability to induce enantioselectivity in catalytic reactions. Compared to similar compounds, it often provides higher yields and better selectivity in asymmetric catalysis.
Properties
Molecular Formula |
C32H62FeP2 |
---|---|
Molecular Weight |
564.6 g/mol |
IUPAC Name |
cyclopentane;ditert-butyl-[1-(2-dicyclohexylphosphanylcyclopentyl)ethyl]phosphane;iron |
InChI |
InChI=1S/C27H52P2.C5H10.Fe/c1-21(29(26(2,3)4)27(5,6)7)24-19-14-20-25(24)28(22-15-10-8-11-16-22)23-17-12-9-13-18-23;1-2-4-5-3-1;/h21-25H,8-20H2,1-7H3;1-5H2; |
InChI Key |
QBLAUUUZOSXQMB-UHFFFAOYSA-N |
Canonical SMILES |
CC(C1CCCC1P(C2CCCCC2)C3CCCCC3)P(C(C)(C)C)C(C)(C)C.C1CCCC1.[Fe] |
Origin of Product |
United States |
Disclaimer and Information on In-Vitro Research Products
Please be aware that all articles and product information presented on BenchChem are intended solely for informational purposes. The products available for purchase on BenchChem are specifically designed for in-vitro studies, which are conducted outside of living organisms. In-vitro studies, derived from the Latin term "in glass," involve experiments performed in controlled laboratory settings using cells or tissues. It is important to note that these products are not categorized as medicines or drugs, and they have not received approval from the FDA for the prevention, treatment, or cure of any medical condition, ailment, or disease. We must emphasize that any form of bodily introduction of these products into humans or animals is strictly prohibited by law. It is essential to adhere to these guidelines to ensure compliance with legal and ethical standards in research and experimentation.