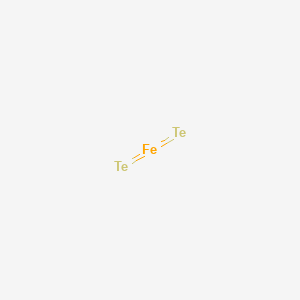
Iron ditelluride
Overview
Description
Iron ditelluride (FeTe₂) is a crystalline transition metal ditelluride belonging to the 3d series. It is known for its high conductivity and unique optothermal properties. This compound has a marcasite structure and exhibits a narrow 3d band, making it a particularly conductive material .
Preparation Methods
Synthetic Routes and Reaction Conditions: Iron ditelluride can be synthesized through various methods, including hydrothermal and chemical vapor deposition techniques. One common method involves the reaction of elemental tellurium with iron (II) complexes in an alkaline aqueous solution at elevated temperatures (around 140°C) under atmospheric pressure . Another method is the hydrothermal coreduction process, which uses a reductant such as ammonium hydroxide to produce this compound nanocrystallites .
Industrial Production Methods: In industrial settings, this compound can be produced by annealing amorphous iron oxide layers, predeposited by spray pyrolysis of iron chloride hexahydrate-based aqueous solution onto heated pyrex glass substrates, under a tellurium-rich atmosphere . This method ensures a good crystalline state with a preferential orientation of the crystallites along the (111) direction.
Chemical Reactions Analysis
Types of Reactions: Iron ditelluride undergoes various chemical reactions, including oxidation, reduction, and substitution reactions.
Common Reagents and Conditions:
Oxidation: this compound can be oxidized using oxidizing agents such as hydrogen peroxide or nitric acid.
Reduction: It can be reduced using reducing agents like sodium borohydride or lithium aluminum hydride.
Substitution: Substitution reactions can occur with halogens or other chalcogenides under appropriate conditions.
Major Products Formed: The major products formed from these reactions depend on the specific reagents and conditions used. For example, oxidation of this compound may produce iron oxides and tellurium oxides, while reduction may yield elemental iron and tellurium .
Scientific Research Applications
Iron ditelluride has a wide range of scientific research applications due to its unique properties:
Chemistry: It is used in the synthesis of various nanomaterials and as a catalyst in chemical reactions.
Biology: this compound’s magnetic properties make it useful in biological imaging and as a contrast agent in magnetic resonance imaging (MRI).
Mechanism of Action
The mechanism by which iron ditelluride exerts its effects is primarily related to its electronic and magnetic properties. The narrow 3d band and high conductivity facilitate efficient electron transfer processes, making it an effective catalyst and electrode material. Additionally, its magnetic properties enable its use in imaging and therapeutic applications .
Comparison with Similar Compounds
- Iron disulfide (FeS₂)
- Iron diselenide (FeSe₂)
- Molybdenum ditelluride (MoTe₂)
- Tungsten ditelluride (WTe₂)
Comparison: Iron ditelluride is unique due to its high conductivity and optothermal properties, which are not as pronounced in its sulfur and selenium analogs. Compared to molybdenum and tungsten ditellurides, this compound has a narrower 3d band, making it more conductive and suitable for specific applications such as high-performance electrodes and catalysts .
Biological Activity
Iron ditelluride (FeTe) is a compound that has garnered interest in various scientific fields, particularly due to its unique properties and potential applications. This article explores the biological activity of this compound, including its interactions at the cellular level, potential toxicity, and implications for health.
Overview of this compound
This compound is a layered compound belonging to the family of iron chalcogenides. It has been studied for its structural, optoelectronic, and superconducting properties. Recent research has also begun to examine its biological implications, particularly concerning its interactions with biological systems and potential toxicity.
The biological activity of this compound can be attributed to its chemical composition and the behavior of tellurium (Te) within biological systems. Tellurium compounds have been shown to exert various toxic effects on both prokaryotic and eukaryotic organisms. The mechanisms include:
- Oxidative Stress : Tellurium can induce oxidative stress by generating reactive oxygen species (ROS), which can damage cellular components such as lipids, proteins, and DNA .
- Enzyme Inhibition : Tellurium compounds can interact with thiol groups in enzymes, leading to inhibition of essential enzymatic activities .
- Cellular Uptake : The uptake of tellurium compounds into cells can disrupt cellular functions, including ATP synthesis, which is crucial for energy metabolism .
Toxicological Studies
Several studies have investigated the toxicological profile of tellurium compounds, including this compound:
- Bacterial Resistance : Research indicates that certain bacteria have developed resistance mechanisms against tellurium compounds by reducing toxic tellurite (TeO) to less harmful elemental tellurium (Te) . This reduction process is facilitated by specific enzymes that detoxify tellurium.
- Cellular Impact : In vitro studies have shown that exposure to tellurium compounds can lead to significant alterations in protein synthesis and cellular respiration in various microbial species .
Study on Tellurium Compounds
A comprehensive study published in 2021 highlighted the effects of tellurium on bacterial cells. The researchers found that tellurite exposure disrupted ATP synthesis in E. coli, leading to reduced growth rates and increased cell death. Additionally, resistant strains exhibited enhanced protein synthesis when exposed to sub-lethal concentrations of tellurite, suggesting a complex interaction between toxicity and resistance mechanisms .
This compound Nanorods for Detection
Another study focused on this compound nanorods (FeTe NRs) as a low-cost system for detecting mercury species. The findings indicated that FeTe NRs could interact specifically with mercury ions, demonstrating potential applications in environmental monitoring and bioremediation . This highlights the dual role of this compound not only as a compound with potential toxicity but also as a tool for detecting harmful substances.
Data Table: Summary of Biological Effects
Properties
IUPAC Name |
bis(tellanylidene)iron | |
---|---|---|
Source | PubChem | |
URL | https://pubchem.ncbi.nlm.nih.gov | |
Description | Data deposited in or computed by PubChem | |
InChI |
InChI=1S/Fe.2Te | |
Source | PubChem | |
URL | https://pubchem.ncbi.nlm.nih.gov | |
Description | Data deposited in or computed by PubChem | |
InChI Key |
NLNGCZOQLAKUDC-UHFFFAOYSA-N | |
Source | PubChem | |
URL | https://pubchem.ncbi.nlm.nih.gov | |
Description | Data deposited in or computed by PubChem | |
Canonical SMILES |
[Fe](=[Te])=[Te] | |
Source | PubChem | |
URL | https://pubchem.ncbi.nlm.nih.gov | |
Description | Data deposited in or computed by PubChem | |
Molecular Formula |
FeTe2 | |
Source | PubChem | |
URL | https://pubchem.ncbi.nlm.nih.gov | |
Description | Data deposited in or computed by PubChem | |
DSSTOX Substance ID |
DTXSID801314301 | |
Record name | Iron telluride (FeTe2) | |
Source | EPA DSSTox | |
URL | https://comptox.epa.gov/dashboard/DTXSID801314301 | |
Description | DSSTox provides a high quality public chemistry resource for supporting improved predictive toxicology. | |
Molecular Weight |
311.0 g/mol | |
Source | PubChem | |
URL | https://pubchem.ncbi.nlm.nih.gov | |
Description | Data deposited in or computed by PubChem | |
CAS No. |
12023-03-9 | |
Record name | Iron telluride (FeTe2) | |
Source | CAS Common Chemistry | |
URL | https://commonchemistry.cas.org/detail?cas_rn=12023-03-9 | |
Description | CAS Common Chemistry is an open community resource for accessing chemical information. Nearly 500,000 chemical substances from CAS REGISTRY cover areas of community interest, including common and frequently regulated chemicals, and those relevant to high school and undergraduate chemistry classes. This chemical information, curated by our expert scientists, is provided in alignment with our mission as a division of the American Chemical Society. | |
Explanation | The data from CAS Common Chemistry is provided under a CC-BY-NC 4.0 license, unless otherwise stated. | |
Record name | Iron telluride (FeTe2) | |
Source | ChemIDplus | |
URL | https://pubchem.ncbi.nlm.nih.gov/substance/?source=chemidplus&sourceid=0012023039 | |
Description | ChemIDplus is a free, web search system that provides access to the structure and nomenclature authority files used for the identification of chemical substances cited in National Library of Medicine (NLM) databases, including the TOXNET system. | |
Record name | Iron telluride (FeTe2) | |
Source | EPA Chemicals under the TSCA | |
URL | https://www.epa.gov/chemicals-under-tsca | |
Description | EPA Chemicals under the Toxic Substances Control Act (TSCA) collection contains information on chemicals and their regulations under TSCA, including non-confidential content from the TSCA Chemical Substance Inventory and Chemical Data Reporting. | |
Record name | Iron telluride (FeTe2) | |
Source | EPA DSSTox | |
URL | https://comptox.epa.gov/dashboard/DTXSID801314301 | |
Description | DSSTox provides a high quality public chemistry resource for supporting improved predictive toxicology. | |
Record name | Iron ditelluride | |
Source | European Chemicals Agency (ECHA) | |
URL | https://echa.europa.eu/substance-information/-/substanceinfo/100.031.508 | |
Description | The European Chemicals Agency (ECHA) is an agency of the European Union which is the driving force among regulatory authorities in implementing the EU's groundbreaking chemicals legislation for the benefit of human health and the environment as well as for innovation and competitiveness. | |
Explanation | Use of the information, documents and data from the ECHA website is subject to the terms and conditions of this Legal Notice, and subject to other binding limitations provided for under applicable law, the information, documents and data made available on the ECHA website may be reproduced, distributed and/or used, totally or in part, for non-commercial purposes provided that ECHA is acknowledged as the source: "Source: European Chemicals Agency, http://echa.europa.eu/". Such acknowledgement must be included in each copy of the material. ECHA permits and encourages organisations and individuals to create links to the ECHA website under the following cumulative conditions: Links can only be made to webpages that provide a link to the Legal Notice page. | |
Disclaimer and Information on In-Vitro Research Products
Please be aware that all articles and product information presented on BenchChem are intended solely for informational purposes. The products available for purchase on BenchChem are specifically designed for in-vitro studies, which are conducted outside of living organisms. In-vitro studies, derived from the Latin term "in glass," involve experiments performed in controlled laboratory settings using cells or tissues. It is important to note that these products are not categorized as medicines or drugs, and they have not received approval from the FDA for the prevention, treatment, or cure of any medical condition, ailment, or disease. We must emphasize that any form of bodily introduction of these products into humans or animals is strictly prohibited by law. It is essential to adhere to these guidelines to ensure compliance with legal and ethical standards in research and experimentation.