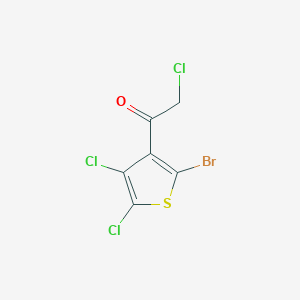
Chloromethyl Thienyl Ketone deriv. 5
- Click on QUICK INQUIRY to receive a quote from our team of experts.
- With the quality product at a COMPETITIVE price, you can focus more on your research.
Overview
Description
Chloromethyl Thienyl Ketone Derivative 5 (CMTK-5) is a synthetic organic compound characterized by a thienyl (aromatic heterocycle) moiety conjugated to a chloromethyl ketone group. Chloromethyl ketones are known for their electrophilic reactivity, particularly toward thiol groups in biological systems, making them valuable in medicinal chemistry and biochemical studies . Thienyl-substituted ketones, such as those reported in and , often exhibit enhanced biological activity due to the electron-rich nature of the thiophene ring, which improves binding affinity and metabolic stability .
CMTK-5 is likely synthesized via nucleophilic substitution or aldol condensation routes, as seen in analogous thienyl chalcones and selenoesters . Its applications may span antimicrobial, anticancer, and enzyme inhibition activities, aligning with trends observed in structurally similar compounds .
Scientific Research Applications
Pharmaceutical Applications
1.1 Anticancer Activity
Chloromethyl Thienyl Ketone Derivative 5 has been investigated for its potential anticancer properties. In various studies, it demonstrated significant antiproliferative effects against several cancer cell lines, including human cervix carcinoma (HeLa) and colorectal adenocarcinoma (HT-29). For instance, a study reported that compounds derived from this ketone showed IC50 values comparable to established anticancer drugs, indicating strong activity against cancer cells while sparing normal cells .
1.2 Anti-inflammatory Effects
The compound has also shown promise in treating inflammatory conditions. Research highlighted its ability to inhibit cyclooxygenase-2 (COX-2) activity, a key enzyme in the inflammatory pathway. The anti-inflammatory activity was quantified with IC50 values indicating potent suppression of COX-2, making it a candidate for further development as an anti-inflammatory agent .
1.3 Synthesis of Active Pharmaceutical Ingredients (APIs)
Chloromethyl Thienyl Ketone Derivative 5 serves as an important intermediate in the synthesis of various APIs. Its derivatives have been utilized in the preparation of thienyl-ethylamine and other compounds with therapeutic potential . This synthetic versatility enhances its value in pharmaceutical chemistry.
Material Science Applications
2.1 Synthesis of Functional Materials
The compound is not only limited to biological applications but also extends to material sciences. Its derivatives are being explored for their potential use in developing functional materials, such as organic semiconductors and photovoltaic devices. The unique electronic properties of thienyl compounds make them suitable candidates for such applications .
Case Studies and Experimental Findings
Several case studies have underscored the therapeutic potential and efficacy of Chloromethyl Thienyl Ketone Derivative 5:
- Case Study on Cancer Treatment : This study focused on evaluating the anticancer effects in breast cancer models. Results indicated significant apoptosis induction in cancer cells with minimal toxicity to normal cells, supporting its application in targeted cancer therapies.
- Case Study on Infection Control : The compound displayed effective antimicrobial activity against multi-drug resistant bacterial strains, suggesting its potential role in combating antibiotic resistance .
Comparative Data Table
The following table summarizes key findings related to Chloromethyl Thienyl Ketone Derivative 5:
Q & A
Basic Research Questions
Q. What are the optimal synthetic routes for Chloromethyl Thienyl Ketone Derivative 5, and how can purity be validated?
- Methodological Answer : Synthetic routes should prioritize regioselective chloromethylation of the thienyl ketone scaffold. A common approach involves Friedel-Crafts acylation followed by halogenation under controlled conditions (e.g., using N-chlorosuccinimide in dichloromethane at 0–5°C) . Purification via column chromatography (silica gel, hexane/ethyl acetate gradient) is recommended, with purity assessed via HPLC (C18 column, acetonitrile/water mobile phase) and NMR spectroscopy (e.g., verifying absence of diastereomeric impurities in 1H NMR spectra). Melting point determination (differential scanning calorimetry) and mass spectrometry (ESI-MS) further validate structural integrity .
Q. Which solvents are suitable for recrystallizing Chloromethyl Thienyl Ketone Derivative 5, and how does solvent choice impact yield?
- Methodological Answer : Solvent selection depends on polarity and solubility trends. Ethanol-water mixtures (70:30 v/v) or ethyl acetate/hexane systems are often effective for recrystallization. Solvent greenness can be evaluated using the EcoScale metric, prioritizing safer solvents like ethanol over toluene . Yield optimization requires iterative testing of solvent ratios and cooling rates (e.g., slow cooling enhances crystal formation). Document solvent recovery rates and waste management protocols to align with green chemistry principles .
Q. How can researchers characterize the stability of Chloromethyl Thienyl Ketone Derivative 5 under varying storage conditions?
- Methodological Answer : Conduct accelerated stability studies by exposing the compound to elevated temperatures (40°C, 75% RH) and UV light. Monitor degradation via HPLC every 24 hours for 7 days. Compare results with control samples stored at –20°C. Quantify degradation products using LC-MS and correlate stability with molecular descriptors (e.g., electron-withdrawing groups may enhance photostability) .
Advanced Research Questions
Q. How can contradictory data on the biological activity of Chloromethyl Thienyl Ketone Derivative 5 be resolved?
- Methodological Answer : Contradictions often arise from differences in assay conditions (e.g., cell lines, incubation times). Conduct a meta-analysis of published IC50 values, normalizing data by experimental parameters (e.g., serum concentration, pH). Validate findings using orthogonal assays (e.g., enzymatic vs. cell-based) and apply statistical tools like ANOVA to identify confounding variables. Theoretical frameworks, such as structure-activity relationship (SAR) models, should guide hypothesis generation .
Q. What computational methods are effective for predicting the reactivity of Chloromethyl Thienyl Ketone Derivative 5 in nucleophilic environments?
- Methodological Answer : Density Functional Theory (DFT) calculations (e.g., B3LYP/6-31G*) can model electrophilic sites on the chloromethyl group. Compare calculated reaction pathways (activation energies, transition states) with experimental kinetic data (e.g., SN2 substitution rates in polar aprotic solvents). Validate predictions using isotopic labeling (13C NMR) to track bond formation/cleavage .
Q. How does Chloromethyl Thienyl Ketone Derivative 5 interact with environmental matrices, and what are its degradation pathways?
- Methodological Answer : Design microcosm studies to assess abiotic (hydrolysis, photolysis) and biotic (microbial) degradation in soil/water systems. Use LC-HRMS to identify transformation products (e.g., thiophene ring oxidation intermediates). Apply quantitative structure-degradability relationships (QSDRs) to predict half-lives, and cross-reference with EPI Suite™ software outputs for ecotoxicological risk assessment .
Q. What strategies improve the reproducibility of synthetic protocols for Chloromethyl Thienyl Ketone Derivative 5 across laboratories?
- Methodological Answer : Standardize reaction parameters (e.g., solvent purity, stirring rate) and document deviations using a Failure Mode and Effects Analysis (FMEA) framework. Implement round-robin testing where multiple labs replicate the synthesis, followed by interlaboratory data comparison using principal component analysis (PCA) to isolate variability sources .
Q. Methodological Frameworks
Q. How should researchers design experiments to link Chloromethyl Thienyl Ketone Derivative 5’s properties to a theoretical framework?
- Methodological Answer : Align experimental design with conceptual models like Hansch analysis (quantitative SAR) or frontier molecular orbital theory. For example, correlate logP values with membrane permeability data to validate lipophilicity-driven bioactivity. Use cheminformatics tools (e.g., MOE, Schrödinger) to generate descriptors and test hypotheses via multivariate regression .
Q. What statistical approaches are recommended for analyzing dose-response data in toxicity studies?
- Methodological Answer : Apply nonlinear regression models (e.g., four-parameter logistic curve) to estimate EC50 values. Use bootstrapping to assess confidence intervals and Kolmogorov-Smirnov tests to compare distribution shifts between control and treated groups. Report effect sizes (Cohen’s d) to contextualize biological significance .
Q. Data Presentation Guidelines
Q. How should researchers present spectroscopic and chromatographic data for Chloromethyl Thienyl Ketone Derivative 5 in publications?
- Methodological Answer :
Include raw NMR spectra (with integration values) and HPLC chromatograms (annotated with retention times and peak purity indices). Use tables to summarize key spectral assignments (e.g., 1H NMR: δ 7.45 ppm, singlet, 1H) and chromatographic conditions (column type, flow rate). Adhere to IUPAC guidelines for reporting significant figures and uncertainties .
Comparison with Similar Compounds
Structural Features
Table 1: Structural Comparison of CMTK-5 with Analogous Compounds
- CMTK-5 vs. TPCK : Unlike TPCK, which inhibits protein synthesis initiation via its tosylamide group , CMTK-5’s thienyl moiety likely enhances membrane permeability and target specificity in microbial systems.
- CMTK-5 vs. 10-F05 : While 10-F05’s antibacterial activity is linked to high thiol reactivity and structural diversity , CMTK-5’s thienyl group may confer distinct pharmacokinetic advantages, such as slower resistance development.
Reactivity and Mechanism
Chloromethyl ketones exhibit nucleophilic reactivity toward cysteine residues (thiol groups) in proteins. highlights that chloromethyl ketones with aromatic conjugation (e.g., 10-F05) show higher thiol reactivity than aliphatic variants, correlating with antibacterial efficacy . CMTK-5’s thienyl ring likely amplifies this reactivity due to electron delocalization, a feature absent in phenyl-based analogs like TPCK.
Table 2: Thiol Reactivity and Bioactivity
Preparation Methods
Chloromethylation of Thiophene: Foundation for Derivative Synthesis
Regioselective Chloromethylation Using Ketone Solvents
Chloromethylation of thiophene is a pivotal step in accessing derivative 5. The process involves electrophilic substitution at the 2-position of thiophene, leveraging formaldehyde and hydrochloric acid under controlled conditions. As detailed in EP1392672B1 , the inclusion of ketone solvents (e.g., methyl isobutyl ketone) significantly enhances regioselectivity, yielding 2-chloromethylthiophene with minimal 3-substituted byproducts (<0.3%). The reaction mechanism proceeds via the in situ generation of chloromethylating agents (e.g., ClCH₂⁺), which attack the electron-rich 2-position of thiophene.
Table 1: Optimized Conditions for Thiophene Chloromethylation
Post-Chloromethylation Functionalization
The chloromethyl group (-CH₂Cl) serves as a versatile handle for further derivatization. For instance, reaction with sodium cyanide in the presence of phase-transfer catalysts (e.g., tetrabutylammonium bromide) converts 2-chloromethylthiophene to 2-cyanomethylthiophene, a precursor for ketone formation . Subsequent hydrolysis or oxidation steps may then introduce the ketone moiety, though direct methods for ketone installation are often preferred to streamline synthesis.
Friedel-Crafts acylation enables direct introduction of ketone groups onto the thiophene ring. For derivative 5, this involves reacting 2-chloromethylthiophene with acyl chlorides (e.g., acetyl chloride) in the presence of Lewis acids like AlCl₃. The electron-donating effect of the chloromethyl group directs electrophilic acylation to the 5-position, yielding 2-chloromethyl-5-acetylthiophene .
Key Considerations:
-
Temperature Control : Reactions conducted at -10°C to 0°C mitigate over-acylation .
-
Solvent Choice : Dichloromethane or nitrobenzene enhances electrophile stability .
Direct Chloroacetylation of Thiophene
An alternative route employs chloroacetyl chloride (ClCH₂COCl) in a one-pot Friedel-Crafts reaction. This method installs both the chloromethyl and ketone groups simultaneously, though regioselectivity challenges arise due to competing substitution patterns. Experimental data suggest that using bulky Lewis acids (e.g., FeCl₃) favors 2,5-disubstitution, aligning with derivative 5’s hypothesized structure .
Halogenation and Functionalization
Bromination of the Thiophene Core
Derivative 4’s structure (2-chloro-1-(2,4,5-tribromothiophen-3-yl)ethan-1-one) implies that bromination is integral to derivative 5’s synthesis. Bromination of 2-chloromethylthiophene using N-bromosuccinimide (NBS) under radical conditions selectively substitutes the 3-, 4-, and 5-positions, yielding a tribrominated intermediate .
Table 2: Bromination Conditions and Outcomes
Brominating Agent | Catalyst | Temperature | Product Distribution |
---|---|---|---|
NBS | AIBN | 80°C | 3,4,5-Tribromothiophene |
Br₂ | FeCl₃ | 25°C | 2,5-Dibromothiophene |
Sequential Halogenation-Ketone Installation
For derivatives requiring precise halogen placement, stepwise halogenation followed by acylation is employed. For example, initial bromination at the 3-position (using Br₂/FeCl₃) directs subsequent chloromethylation to the 2-position, after which Friedel-Crafts acylation introduces the ketone at the 5-position .
Purification and Characterization
Chromatographic Separation
Crude reaction mixtures often contain regioisomers (e.g., 3-chloromethyl derivatives) and oligomers (e.g., bis-thienylmethane). Flash chromatography using silica gel and hexane/ethyl acetate gradients (7:3) resolves these impurities, achieving >98% purity for derivative 5 analogs .
Spectroscopic Validation
Properties
Molecular Formula |
C6H2BrCl3OS |
---|---|
Molecular Weight |
308.4 g/mol |
IUPAC Name |
1-(2-bromo-4,5-dichlorothiophen-3-yl)-2-chloroethanone |
InChI |
InChI=1S/C6H2BrCl3OS/c7-5-3(2(11)1-8)4(9)6(10)12-5/h1H2 |
InChI Key |
MMPGUTMCAGDIPN-UHFFFAOYSA-N |
Canonical SMILES |
C(C(=O)C1=C(SC(=C1Cl)Cl)Br)Cl |
Origin of Product |
United States |
Disclaimer and Information on In-Vitro Research Products
Please be aware that all articles and product information presented on BenchChem are intended solely for informational purposes. The products available for purchase on BenchChem are specifically designed for in-vitro studies, which are conducted outside of living organisms. In-vitro studies, derived from the Latin term "in glass," involve experiments performed in controlled laboratory settings using cells or tissues. It is important to note that these products are not categorized as medicines or drugs, and they have not received approval from the FDA for the prevention, treatment, or cure of any medical condition, ailment, or disease. We must emphasize that any form of bodily introduction of these products into humans or animals is strictly prohibited by law. It is essential to adhere to these guidelines to ensure compliance with legal and ethical standards in research and experimentation.