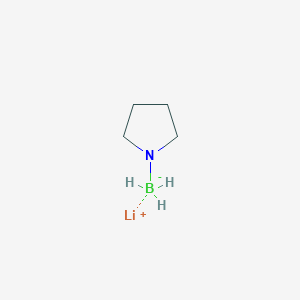
Lithium pyrrolidinoborohydride
- Click on QUICK INQUIRY to receive a quote from our team of experts.
- With the quality product at a COMPETITIVE price, you can focus more on your research.
Overview
Description
Lithium pyrrolidinoborohydride (LiBH₃NR₂, where NR₂ = pyrrolidino; CAS 144188-76-1) is a specialized reducing agent in organic synthesis, belonging to the class of lithium aminoborohydrides (LABs). It is typically supplied as a 1 M solution in tetrahydrofuran (THF) and has a molecular weight of 90.89 g/mol . This reagent is widely employed for selective reductions, particularly in tandem amination-reduction reactions and controlled cleavage of amides. Its pyrrolidino substituent confers moderate steric bulk, enabling distinct reactivity compared to other LABs .
Scientific Research Applications
Chemical Properties and Mechanism of Action
Chemical Structure :
- Molecular Formula : C4H8BLiN
- Molecular Weight : 87.9 g/mol
- CAS Number : 144188-76-1
Lithium pyrrolidinoborohydride operates primarily through a reduction-amination reaction , effectively reducing carbonyl and carboxylic acid derivatives to their corresponding alcohols. Its mechanism involves nucleophilic attack on electrophilic centers, leading to the transformation of various functional groups.
Key Applications
-
Reduction of Carbonyl Compounds :
- This compound is widely used to convert ketones and aldehydes into their respective alcohols. This transformation is crucial in organic synthesis for the preparation of alcohols from more oxidized precursors.
- Case Study : In a study, this compound was employed to reduce cyclopentenones with high regioselectivity, yielding alcohols with minimal side reactions .
- Reduction of Amides :
- Selective Reduction in Complex Mixtures :
-
Reduction of Imines and Other Functional Groups :
- The compound also facilitates the reduction of imines to amines under mild conditions, expanding its utility in synthesizing nitrogen-containing compounds.
- It has been shown to effectively reduce epoxides, oximes, nitriles, and halides while demonstrating limited reactivity towards carboxylic acids .
Summary of Biological Activities
The following table summarizes the various reactions facilitated by this compound:
Reaction Type | Substrate Type | Product Type | Yield (%) | Conditions |
---|---|---|---|---|
Reduction of Amides | Amides | Alcohols | >90 | THF, Room Temperature |
Reduction of Ketones | Ketones | Alcohols | 94 | THF at 0 °C |
Reduction of Imines | Imines | Amines | Varies | Mild Conditions |
Reduction of Epoxides | Epoxides | Alcohols | High | THF at Ambient Temperature |
Reduction of Nitriles | Nitriles | Amines | High | THF at Ambient Temperature |
Q & A
Basic Research Questions
Q. What are the standard synthetic protocols and characterization methods for lithium pyrrolidinoborohydride (LiBHNR₂)?
this compound is typically synthesized by reacting lithium borohydride with pyrrolidine in tetrahydrofuran (THF) under inert conditions. Characterization involves nuclear magnetic resonance (NMR) spectroscopy (¹¹B and ¹H) to confirm borohydride coordination and purity. Quantitative analysis of active hydride content is performed via gas chromatography (GC) or titration against standardized acids. Always validate purity (>95%) before use in sensitive reactions .
Q. What safety measures are critical when handling this compound in laboratory settings?
Due to its pyrophoric nature and reactivity with moisture, strict adherence to SOPs is required:
- Use glove boxes or Schlenk lines under nitrogen/argon atmospheres.
- Equip labs with flame-resistant materials and Class D fire extinguishers.
- Train personnel on emergency procedures for spills (e.g., smothering with sand) and document compliance with institutional safety protocols .
Q. What reaction mechanisms are associated with this compound in tandem amination-reduction processes?
LiBHNR₂ facilitates nucleophilic aromatic substitution followed by nitrile reduction. For example, 2-fluorobenzonitrile reacts via displacement of fluoride by pyrrolidine, forming an intermediate imine, which is reduced to 2-(pyrrolidino)benzylamine. Mechanistic studies use kinetic isotopic effects (KIEs) and DFT calculations to validate stepwise vs. concerted pathways .
Advanced Research Questions
Q. How can reaction conditions be optimized to improve selectivity in LiBHNR₂-mediated tandem amination-reduction?
Key variables include:
- Solvent polarity : THF vs. diglyme (higher polarity accelerates nucleophilic substitution).
- Temperature : Reflux conditions (66–68°C in THF) balance reaction rate and by-product formation.
- Stoichiometry : Excess LiBHNR₂ (1.5–2.0 eq.) minimizes incomplete reduction. Systematic Design of Experiments (DoE) matrices are recommended to identify optimal parameters .
Q. What analytical strategies resolve contradictory product distributions in LiBHNR₂ reactions with halogenated benzonitriles?
Competing pathways (e.g., direct reduction vs. substitution-reduction) produce mixtures like 70:30 ratios of 2-(pyrrolidino)benzylamine and 2-chlorobenzylamine. Use GC-MS or HPLC coupled with kinetic studies to track intermediate formation. Adjusting steric bulk of the amine (e.g., morpholine vs. pyrrolidine) can shift selectivity .
Q. How does LiBHNR₂ compare to other lithium aminoborohydrides (LABs) in substrate scope and efficiency?
Comparative studies show LiBHNR₂ outperforms less nucleophilic LABs (e.g., lithium morpholinoborohydride) in reactions requiring strong nucleophilic activation. For example, 2-fluorobenzonitrile achieves 84% yield with LiBHNR₂ vs. 65% with lithium dimethylaminoborohydride. Substrate screening across electron-deficient aromatics (e.g., nitro-, cyano-substituted) reveals scope limitations .
Q. What computational methods predict LiBHNR₂ reactivity in novel reaction systems?
Density Functional Theory (DFT) models simulate transition states for substitution and reduction steps. Parameters like Fukui indices identify electrophilic centers in substrates. Pair computational insights with experimental validation (e.g., Hammett plots) to design new applications, such as asymmetric reductions .
Q. How can by-products from LiBHNR₂ reactions be minimized or repurposed?
Side products (e.g., unreacted nitriles) are separable via column chromatography or recrystallization. Catalytic additives (e.g., Pd/C) or scavengers (molecular sieves) mitigate moisture-induced decomposition. Green chemistry approaches, such as solvent recycling, improve sustainability .
Q. Methodological Frameworks for Research Design
Q. What frameworks (e.g., PICOT, FINER) guide hypothesis formulation for LiBHNR₂ studies?
- PICOT : Define Population (substrate class), Intervention (LiBHNR₂ conditions), Comparison (alternative reagents), Outcome (yield/selectivity), Time (reaction duration).
- FINER : Ensure questions are Feasible (lab resources), Interesting (novel mechanisms), Novel (unexplored substrates), Ethical (safety compliance), Relevant (organic synthesis trends) .
Q. How should researchers address data contradictions in kinetic studies of LiBHNR₂?
Apply statistical tools (ANOVA, regression analysis) to isolate variables causing discrepancies. Replicate experiments under controlled humidity/temperature. Publish negative results to inform community-wide reproducibility efforts .
Comparison with Similar Compounds
Comparative Analysis with Similar Compounds
Structural and Molecular Properties
The table below summarizes key structural and functional differences between lithium pyrrolidinoborohydride and related borohydrides:
Compound | Molecular Formula | Molecular Weight (g/mol) | CAS Number | Key Substituent | Steric Bulk |
---|---|---|---|---|---|
This compound | C₄H₁₁BLiN | 90.89 | 144188-76-1 | Pyrrolidino | Moderate |
Lithium morpholinoborohydride | C₄H₁₃BLiNO | 106.89 | N/A | Morpholino | Low |
Lithium diisopropylaminoborohydride | C₆H₁₈BLiN | 135.95 | N/A | Diisopropylamino | High |
Lithium borohydride (LiBH₄) | LiBH₄ | 21.78 | 16949-15-8 | None | Minimal |
Reactivity and Selectivity in Key Reactions
Tandem Amination-Reduction of Halobenzonitriles
This compound demonstrates high efficiency in substituting halogens with amines while reducing nitriles to benzylamines. For example:
- Reaction with 2-fluorobenzonitrile yields 84% of 2-(pyrrolidino)benzylamine .
- Similar reactions with 4-fluorobenzonitrile achieve 89% yield .
In contrast, lithium morpholinoborohydride (lower steric bulk) shows comparable yields but may favor different amine products due to the morpholino group’s electronic effects. Bulkier LABs like lithium diisopropylaminoborohydride exhibit reduced reactivity in such substitutions due to steric hindrance .
Controlled Reduction of Amides
This compound’s moderate steric profile enables selective C–O bond cleavage in tertiary amides, producing alcohols as major products. For example:
In comparison, lithium diisopropylaminoborohydride (higher steric bulk) favors C–N bond cleavage, yielding amines instead . Lithium borohydride (LiBH₄), lacking directional steric control, often results in unselective reductions .
Reaction with 2-Chlorobenzonitrile
A critical distinction arises in halogen substitution:
- This compound produces a 70:30 mixture of 2-(pyrrolidino)benzylamine and 2-chlorobenzylamine .
- LiBH₄NMe₂ (dimethylamino variant) yields a similar ratio but with dimethylamino products, highlighting the role of the amine group in directing substitution .
Key Research Findings
- Steric Tuning: The steric bulk of LABs dictates reaction pathways. Pyrrolidinoborohydride balances reactivity and selectivity, making it ideal for applications requiring moderate steric control .
- Nitrogen Transfer: Pyrrolidinoborohydride efficiently transfers its pyrrolidino group to aromatic systems, enabling synthesis of complex amines .
- Yield Superiority: In fluorobenzonitrile reductions, pyrrolidinoborohydride outperforms traditional reagents like NaBH₄ in both yield and selectivity .
Preparation Methods
Synthetic Pathway and Reaction Mechanism
Formation of Pyrrolidine-Borane Complex
The initial step involves the exothermic reaction between pyrrolidine (C₄H₈NH) and BH₃·THF at ambient temperature. The boron atom in BH₃ coordinates with the lone pair of electrons on pyrrolidine’s nitrogen, forming a stable amine-borane adduct (H₃B·NC₄H₈). This intermediate is characterized by its trigonal planar geometry around boron, as confirmed by ¹¹B-NMR spectroscopy (δ = -23 ppm, quartet) .
Key Parameters:
-
Molar Ratio: A 1:1 stoichiometry of pyrrolidine to BH₃·THF is critical to avoid unreacted starting materials.
-
Solvent: THF serves as both solvent and stabilizing agent for BH₃.
-
Temperature: Conducted at 25°C, with cooling to 0°C required for subsequent deprotonation .
Deprotonation with Organolithium Reagents
The amine-borane adduct undergoes deprotonation using n-BuLi in anhydrous THF. This step generates the lithium aminoborohydride species through abstraction of the N–H proton, yielding Li⁺[H₂B·NC₄H₈]⁻. The reaction is quantitative when performed at 0–10°C over 2–3 hours, as monitored by hydride titration .
Reaction Conditions:
-
Base Strength: n-BuLi (pKa ~50) is sufficiently strong to deprotonate the amine-borane complex (pKa ~25–30).
-
Solvent System: THF/hexane mixtures enhance solubility of the lithium species while minimizing side reactions.
-
Yield: 92% purity confirmed via ¹H-NMR and hydride activity assays .
Experimental Protocols and Optimization
Standard Laboratory-Scale Synthesis
A representative procedure from U.S. Patent 5,466,798 involves :
-
Charge Pyrrolidine: Add pyrrolidine (210 mmol) to a nitrogen-flushed reactor.
-
Introduce BH₃·THF: Slowly add BH₃·THF (1M, 210 mL) with stirring at 25°C for 1 hour.
-
Cool and Deprotonate: Cool to 0°C, then add n-BuLi (2.5M, 231 mmol) dropwise. Stir for 2–3 hours.
-
Warm to Ambient Temperature: Allow the mixture to reach 25°C, stirring for an additional hour.
-
Analysis: Determine yield via hydride titration (4.0M hydride concentration) and ¹¹B-NMR.
Critical Considerations:
-
Moisture Sensitivity: All steps require strict exclusion of moisture to prevent hydrolysis.
-
Exotherm Management: Slow addition of n-BuLi prevents thermal decomposition.
Scalability and Industrial Adaptations
For large-scale production, the reaction is conducted in hydrocarbon/THF mixtures (e.g., toluene/THF) to improve handling. Patent US20030187164A1 highlights that lithium pyrrolidine derivatives exhibit enhanced stability in THF/hydrocarbon solutions (e.g., 16.6% Li-pyrrolidine in toluene/THF), with no significant decomposition after 16 days at 20°C .
Stability Data:
Storage Temperature (°C) | Active Base After 16 Days (mmol/g) |
---|---|
0 | 2.22 |
20 | 2.21 |
40 | 1.91 |
Comparative Analysis of Aminoborohydride Synthesis
Table 1 synthesizes data from U.S. Patent 5,466,798, demonstrating the generality of the method for related lithium aminoborohydrides :
Table 1: ¹¹B-NMR Chemical Shifts of Select Lithium Aminoborohydrides
Amine Reactant | Amine-Borane δ (ppm) | Lithium Aminoborohydride δ (ppm) |
---|---|---|
Dimethylamine | -18 (q) | -17 (q) |
Diethylamine | -23 (q) | -16 (q) |
Piperidine | -18 (q) | -20 (q) |
Morpholine | -16 (q) | -16 (q) |
Key Observations:
-
Steric Effects: Bulky amines (e.g., diisopropylamine) yield less stable borohydrides due to hindered boron coordination.
-
Electron Donation: Electron-rich amines (e.g., pyrrolidine) enhance hydride donor capacity, as evidenced by lower δ values in ¹¹B-NMR .
Characterization and Quality Control
Spectroscopic Methods
-
¹¹B-NMR: Primary tool for confirming boron environment. LiPyrBH₃ exhibits a quartet at δ = -23 ppm (J = 80 Hz), indicative of three equivalent hydrogens bonded to boron .
-
¹H-NMR: Protons on the pyrrolidine ring appear as multiplet signals between δ 1.6–2.4 ppm, while BH₃ hydrogens resonate as a broad singlet at δ 3.2 ppm .
Hydride Titration
Hydride content is quantified via hydrolysis with 3M HCl, measuring evolved hydrogen gas. Commercial batches typically assay at 4.0M hydride concentration (±5%) .
Applications in Organic Synthesis
LiPyrBH₃ reduces esters, lactones, and amides at 25°C without attacking carboxylic acids. Example reductions include:
Properties
Molecular Formula |
C4H11BLiN |
---|---|
Molecular Weight |
90.9 g/mol |
IUPAC Name |
lithium;pyrrolidin-1-ylboranuide |
InChI |
InChI=1S/C4H11BN.Li/c5-6-3-1-2-4-6;/h1-4H2,5H3;/q-1;+1 |
InChI Key |
CUBZYJJJUFGOBJ-UHFFFAOYSA-N |
Canonical SMILES |
[Li+].[BH3-]N1CCCC1 |
Origin of Product |
United States |
Disclaimer and Information on In-Vitro Research Products
Please be aware that all articles and product information presented on BenchChem are intended solely for informational purposes. The products available for purchase on BenchChem are specifically designed for in-vitro studies, which are conducted outside of living organisms. In-vitro studies, derived from the Latin term "in glass," involve experiments performed in controlled laboratory settings using cells or tissues. It is important to note that these products are not categorized as medicines or drugs, and they have not received approval from the FDA for the prevention, treatment, or cure of any medical condition, ailment, or disease. We must emphasize that any form of bodily introduction of these products into humans or animals is strictly prohibited by law. It is essential to adhere to these guidelines to ensure compliance with legal and ethical standards in research and experimentation.