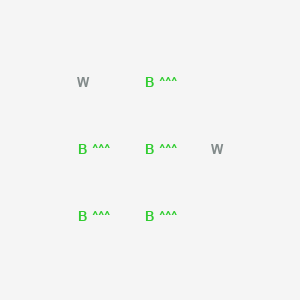
Tungsten boride (W2B5)
Overview
Description
Tungsten boride (W₂B₅) is a boron-rich compound notable for its exceptional hardness, thermal stability, and neutron absorption capabilities. Synthesized via methods like self-propagating high-temperature synthesis (SHS) or spark plasma sintering (SPS), W₂B₅ exhibits a pseudohexagonal or orthorhombic crystal structure (space group Pnnm), depending on synthesis conditions . Its mechanical properties include a Vickers hardness of 25–27 GPa and fracture toughness of ~3–4 MPa√m, making it suitable for drilling tools, wear-resistant coatings, and neutron shielding . W₂B₅ also demonstrates a neutron absorption cross-section of 2.14×10⁻¹ barn, outperforming boron carbide (B₄C) in nuclear applications .
Preparation Methods
Synthetic Routes and Reaction Conditions: Tungsten boride (W2B5) can be synthesized through several methods:
Mechanochemical Synthesis: This method involves the reaction of tungsten dioxide (WO2.72), boron trioxide (B2O3), and magnesium (Mg) powders.
Solid-State Reaction: Tungsten and amorphous boron powders are mixed and treated at high temperatures (800 to 1550°C) in an argon atmosphere.
Industrial Production Methods: Industrial production of tungsten boride typically involves high-temperature reactions and metallothermic reduction processes. These methods ensure the production of tungsten boride with high purity and desired particle sizes .
Types of Reactions:
Oxidation: Tungsten boride can undergo oxidation at high temperatures, forming tungsten oxides and boron oxides.
Reduction: At high temperatures, tungsten boride can be reduced back to its constituent elements, tungsten and boron.
Substitution: Tungsten boride can participate in substitution reactions where boron atoms are replaced by other elements, altering its properties.
Common Reagents and Conditions:
Oxidation: Typically involves exposure to oxygen or air at elevated temperatures.
Reduction: Requires high temperatures and reducing agents such as hydrogen or carbon.
Substitution: Involves the use of other elements or compounds that can replace boron atoms under specific conditions.
Major Products Formed:
Oxidation: Tungsten oxides (WO3) and boron oxides (B2O3).
Reduction: Elemental tungsten and boron.
Substitution: Modified tungsten borides with altered properties.
Scientific Research Applications
Catalysis
W₂B₅ has been utilized as a catalyst in several chemical reactions. Its ability to facilitate hydrogen evolution reactions makes it a candidate for energy-related applications, particularly in fuel cells and hydrogen production technologies. Studies have shown that tungsten boride can enhance reaction rates due to its unique electronic properties and surface characteristics.
Electrochemistry
In electrochemical applications, W₂B₅ is investigated for use as electrodes in batteries and fuel cells. Its excellent electrical conductivity and stability under operational conditions contribute to improved performance metrics in energy storage systems. The compound's ability to withstand harsh electrochemical environments makes it a viable option for next-generation energy devices.
Materials Science
W₂B₅ serves as an additive in composite materials to enhance mechanical properties such as wear resistance and hardness. Research indicates that incorporating W₂B₅ into materials like boron carbide (B₄C) significantly improves their performance. For instance, adding 10 wt% W₂B₅ to B₄C has been shown to refine grain size and enhance mechanical properties like fracture toughness.
High-Temperature Applications
Due to its high melting point and thermal stability, W₂B₅ is employed in high-temperature applications such as crucibles for metallurgy and thermal spraying powders. Its ability to maintain structural integrity at elevated temperatures makes it ideal for processing non-ferrous metals and other materials requiring high thermal resistance.
Nuclear Industry
W₂B₅ is being explored for use in the nuclear industry, particularly as a shielding material in fission and fusion reactors. Its density and thermal properties provide effective radiation shielding capabilities, making it suitable for advanced reactor designs .
Case Study 1: Enhanced Mechanical Properties in Composites
A study demonstrated that the addition of tungsten boride to carbon composites significantly improved wear resistance against steel bearings. The research highlighted how W₂B₅ contributed to enhanced mechanical strength through its hardening effects, making these composites suitable for demanding industrial applications.
Case Study 2: Electrochemical Performance Improvement
Research focusing on the use of W₂B₅ as an electrode material revealed substantial improvements in charge-discharge cycles in lithium-ion batteries. The study noted that the incorporation of tungsten boride led to increased conductivity and reduced degradation over time, indicating its potential for long-lasting energy storage solutions.
Case Study 3: Nuclear Reactor Shielding
In experimental setups simulating fusion reactor conditions, W₂B₅ was evaluated for its effectiveness as a neutron shielding material. Results indicated that the compound not only provided adequate shielding but also maintained structural integrity under high radiation exposure, showcasing its potential role in future nuclear technologies .
Mechanism of Action
The mechanism by which tungsten boride exerts its effects is primarily related to its unique crystal structure and bonding characteristics. The hybridization between the d orbitals of tungsten and the sp orbitals of boron contributes to its exceptional hardness and stability . These interactions also play a crucial role in its catalytic and electrochemical properties.
Comparison with Similar Compounds
Structural and Compositional Comparison
Notes:
- W₂B₅’s structure is debated: reports orthorhombic (Pnnm), while suggests hexagonal, likely due to synthesis variations .
- WB₄’s metastability complicates its industrial use compared to W₂B₅ .
Mechanical and Thermal Properties
Key Findings :
- WB₄ and WB₂ surpass W₂B₅ in hardness but are harder to synthesize .
- Composites like B₄C–W₂B₅ achieve superior toughness (11.9 MPa√m) due to W₂B₅ platelet reinforcement .
Performance Insights :
- W₂B₅ reduces neutron flux in fusion reactors by ~10× compared to pure tungsten .
- Composite materials with W₂B₅ optimize both neutron absorption and mechanical resilience .
Optimized Conditions for W₂B₅ :
Ternary Borides and Composites
Biological Activity
Tungsten boride (W₂B₅) is a compound that has garnered attention not only for its mechanical properties but also for its potential biological applications. This article explores the biological activity of W₂B₅, focusing on its synthesis, properties, and implications in various fields, particularly in biomedicine and environmental science.
Synthesis of Tungsten Boride
The synthesis of W₂B₅ typically involves high-temperature reactions between tungsten trioxide (WO₃) and boron oxide (B₂O₃) or other boron sources. Various methods, including self-propagating high-temperature synthesis (SHS), have been employed to produce tungsten borides with high purity levels. For instance, a study reported achieving over 98% purity of W₂B₅ through a double SHS method, which involved a two-step reaction process followed by acid leaching to remove impurities .
Properties of Tungsten Boride
W₂B₅ exhibits several notable properties that contribute to its potential biological applications:
- Mechanical Strength : With a Vickers hardness of approximately 30 GPa, W₂B₅ is one of the hardest materials known, making it suitable for wear-resistant applications.
- Thermal Stability : It has a high melting point (around 2670 °C), which allows it to maintain structural integrity under extreme conditions.
- Chemical Inertness : The compound is chemically stable and resistant to oxidation, which is advantageous in biological environments where reactivity can lead to adverse effects.
Antimicrobial Properties
Recent studies have begun to investigate the antimicrobial properties of tungsten borides. The unique surface characteristics of W₂B₅ may enhance its interaction with microbial cells, potentially leading to bactericidal effects. Research indicates that metal borides can disrupt bacterial cell membranes, leading to cell death. This property could be harnessed in coatings for medical devices or surfaces in healthcare settings to reduce infection rates.
Biocompatibility
The biocompatibility of W₂B₅ is crucial for its application in biomedical fields. Preliminary studies suggest that tungsten borides may exhibit favorable interactions with biological tissues. For instance, coatings made from tungsten borides have shown reduced cytotoxicity compared to traditional metal coatings, indicating potential for use in implants or prosthetics .
Case Studies
- Biomedical Applications : A study explored the use of W₂B₅ coatings on titanium implants. The results demonstrated improved resistance to wear and corrosion compared to uncoated titanium, suggesting that W₂B₅ can enhance the longevity and performance of implants in vivo .
- Environmental Applications : In environmental catalysis, W₂B₅ has been investigated for its ability to catalyze reactions such as carbon monoxide oxidation. Its stability and catalytic properties make it a candidate for reducing harmful emissions from industrial processes .
Comparative Analysis
The following table summarizes the key properties of tungsten boride (W₂B₅) compared to other transition metal borides:
Compound | Vickers Hardness (GPa) | Melting Point (°C) | Notable Properties |
---|---|---|---|
Tungsten Boride (W₂B₅) | ~30 | 2670 | High hardness, excellent thermal stability |
Molybdenum Boride (Mo₂B₅) | ~20 | 2600 | Lower hardness than tungsten boride |
Titanium Boride (TiB₂) | ~30 | 2980 | Excellent wear resistance |
Zirconium Boride (ZrB₂) | ~25 | 3250 | High melting point |
Chemical Reactions Analysis
Solid-State Reaction with Magnesium Reduction
A two-step self-propagating high-temperature synthesis (SHS) method produces W₂B₅ from tungsten trioxide (WO₃) and boron oxide (B₂O₃):
Step 1 :
Step 2 :
Acid leaching with HCl removes MgO and Mg₃B₂O₆, yielding a product with 98.2 wt.% W₂B₅ purity .
Oxidation Reactions
W₂B₅ oxidizes exothermically in air at elevated temperatures:
Key experimental observations :
-
Weight gain : Theoretical (151.2%) vs. observed (144.3%) due to WO₃ and B₂O₃ evaporation above 800°C .
-
Thermal stability : Oxidation begins at 294°C, peaking at 1134°C with rapid mass loss .
Reduction Reactions
W₂B₅ undergoes reduction in hydrogen or carbon environments:
Conditions :
Environmental Influences on Reactivity
Table 2: Oxidation Reaction Metrics
Reaction Byproducts and Mitigation
Industrial Implications
Q & A
Q. Basic: What established synthesis methods ensure phase-pure W₂B₅, and how do process parameters influence structural outcomes?
Synthesizing phase-pure W₂B₅ requires precise control of stoichiometry and temperature. Common methods include:
- Solid-state reaction : Tungsten and boron powders are heated at 1,500–1,800°C under inert gas. Excess boron is often used to compensate for volatility, but this risks forming secondary phases like WB or W₂B .
- Chemical vapor deposition (CVD) : Gas-phase precursors (e.g., WF₆ and BCl₃) deposit W₂B₅ thin films at lower temperatures (~800°C), minimizing contamination .
Key parameters : - Temperature : Higher temperatures favor densification but may promote grain growth, affecting mechanical properties.
- Boron ratio : A 5:2 B:W molar ratio minimizes secondary phases but requires post-synthesis XRD validation .
Q. Advanced: How can researchers resolve contradictions in reported hardness values (20–45 GPa) for W₂B₅?
Discrepancies arise from variations in:
- Testing methods : Nanoindentation (localized measurement) vs. Vickers hardness (bulk average). Nanoindentation is sensitive to crystallographic orientation and defects .
- Microstructure : Grain boundaries and porosity reduce measured hardness. Use TEM to correlate microstructure with mechanical data (e.g., dislocation density in single-crystal vs. polycrystalline samples) .
Methodological recommendations : - Standardize testing protocols (e.g., ISO 14577 for nanoindentation).
- Report grain size, porosity, and crystallographic orientation in supplementary data .
Q. Basic: What characterization techniques are essential for validating W₂B₅’s structural and compositional integrity?
- X-ray diffraction (XRD) : Confirm phase purity by matching peaks with reference data (e.g., ICDD PDF 00-034-1036 for W₂B₅) .
- Scanning/Transmission Electron Microscopy (SEM/TEM) : Assess grain morphology and detect amorphous regions. Combine with EDS for elemental mapping to verify stoichiometry .
- X-ray photoelectron spectroscopy (XPS) : Identify surface oxidation (e.g., WO₃ formation) that may skew bulk property measurements .
Q. Advanced: How can density functional theory (DFT) models improve predictions of W₂B₅’s stability under irradiation?
DFT simulations address gaps in experimental data by:
- Predicting defect formation energies : Calculate vacancy/interstitial formation under neutron irradiation to identify stable configurations .
- Thermodynamic stability : Compare total energies of W₂B₅ with competing phases (e.g., WB₄) at varying pressures/temperatures .
Validation : Pair computational results with in situ XRD during irradiation experiments to monitor phase transitions .
Q. Basic: What experimental design considerations are critical for measuring W₂B₅’s thermal conductivity in fusion-relevant environments?
- Sample geometry : Use thin films or small pellets to minimize thermal gradient errors.
- Atmosphere control : Conduct tests under high vacuum or inert gas to prevent oxidation.
- Techniques : Laser flash analysis (LFA) for bulk samples; time-domain thermoreflectance (TDTR) for thin films .
Data reporting : Include temperature-dependent conductivity curves and annealing history in supplementary materials .
Q. Advanced: How does boron content optimization enhance W₂B₅’s irradiation resistance in nuclear fusion applications?
- Alloy design : Increase boron content to form boron-rich phases (e.g., W₂B₅ vs. WB), which absorb neutron-induced defects more effectively .
- Experimental validation : Expose samples to proton/ion irradiation and analyze defect clusters via TEM. Compare with unalloyed tungsten to quantify improvement .
Key metric : Displacement-per-atom (DPA) thresholds before amorphization or cracking .
Q. Basic: What are the best practices for reporting W₂B₅ synthesis protocols in academic publications?
- Detailed methodology : Specify precursor purity, heating rates, and gas flow rates.
- Data tables : Include phase composition (XRD), elemental ratios (EDS), and density measurements. Example:
Synthesis Method | Temperature (°C) | B:W Ratio | Phase Purity (XRD) | Hardness (GPa) |
---|---|---|---|---|
Solid-state | 1,600 | 5.5:2 | W₂B₅ (95%) | 38 ± 2 |
CVD | 800 | 5:2 | W₂B₅ (99%) | 42 ± 3 |
- Supplemental files : Provide raw XRD spectra and TEM micrographs .
Q. Advanced: How can synchrotron-based techniques address limitations in conventional W₂B₅ characterization?
- High-resolution XRD : Resolve subtle peak shifts indicative of lattice strain or doping effects.
- X-ray absorption spectroscopy (XAS) : Probe local boron coordination environments to identify amorphous phases .
Application example : Synchrotron XRD revealed a 0.3% lattice expansion in irradiated W₂B₅, linked to vacancy clustering .
Q. Basic: What safety protocols are essential when handling tungsten boride in laboratory settings?
- Ventilation : Use fume hoods during synthesis to mitigate exposure to boron-containing gases (e.g., BCl₃).
- Personal protective equipment (PPE) : Wear nitrile gloves and particulate respirators to prevent inhalation of fine powders .
Q. Advanced: What strategies improve reproducibility in W₂B₅ research across different laboratories?
- Interlaboratory studies : Share reference samples (e.g., pre-synthesized W₂B₅ pellets) to calibrate equipment.
- Open data : Publish raw datasets (XRD, mechanical tests) in repositories like Zenodo for cross-validation .
Example initiative : The Skoltech-Gazpromneft collaboration established standardized protocols for hardness testing, reducing inter-lab variability by 15% .
Properties
InChI |
InChI=1S/5B.2W | |
---|---|---|
Source | PubChem | |
URL | https://pubchem.ncbi.nlm.nih.gov | |
Description | Data deposited in or computed by PubChem | |
InChI Key |
CXOKLXRCTOUPEV-UHFFFAOYSA-N | |
Source | PubChem | |
URL | https://pubchem.ncbi.nlm.nih.gov | |
Description | Data deposited in or computed by PubChem | |
Canonical SMILES |
[B].[B].[B].[B].[B].[W].[W] | |
Source | PubChem | |
URL | https://pubchem.ncbi.nlm.nih.gov | |
Description | Data deposited in or computed by PubChem | |
Molecular Formula |
B5W2 | |
Source | PubChem | |
URL | https://pubchem.ncbi.nlm.nih.gov | |
Description | Data deposited in or computed by PubChem | |
DSSTOX Substance ID |
DTXSID00422824 | |
Record name | Tungsten boride (W2B5) | |
Source | EPA DSSTox | |
URL | https://comptox.epa.gov/dashboard/DTXSID00422824 | |
Description | DSSTox provides a high quality public chemistry resource for supporting improved predictive toxicology. | |
Molecular Weight |
421.7 g/mol | |
Source | PubChem | |
URL | https://pubchem.ncbi.nlm.nih.gov | |
Description | Data deposited in or computed by PubChem | |
CAS No. |
12007-98-6, 149027-54-3 | |
Record name | Tungsten boride (W2B5) | |
Source | ChemIDplus | |
URL | https://pubchem.ncbi.nlm.nih.gov/substance/?source=chemidplus&sourceid=0012007986 | |
Description | ChemIDplus is a free, web search system that provides access to the structure and nomenclature authority files used for the identification of chemical substances cited in National Library of Medicine (NLM) databases, including the TOXNET system. | |
Record name | Tungsten boride (W2B5) | |
Source | EPA Chemicals under the TSCA | |
URL | https://www.epa.gov/chemicals-under-tsca | |
Description | EPA Chemicals under the Toxic Substances Control Act (TSCA) collection contains information on chemicals and their regulations under TSCA, including non-confidential content from the TSCA Chemical Substance Inventory and Chemical Data Reporting. | |
Record name | Tungsten boride (W2B5) | |
Source | EPA DSSTox | |
URL | https://comptox.epa.gov/dashboard/DTXSID00422824 | |
Description | DSSTox provides a high quality public chemistry resource for supporting improved predictive toxicology. | |
Disclaimer and Information on In-Vitro Research Products
Please be aware that all articles and product information presented on BenchChem are intended solely for informational purposes. The products available for purchase on BenchChem are specifically designed for in-vitro studies, which are conducted outside of living organisms. In-vitro studies, derived from the Latin term "in glass," involve experiments performed in controlled laboratory settings using cells or tissues. It is important to note that these products are not categorized as medicines or drugs, and they have not received approval from the FDA for the prevention, treatment, or cure of any medical condition, ailment, or disease. We must emphasize that any form of bodily introduction of these products into humans or animals is strictly prohibited by law. It is essential to adhere to these guidelines to ensure compliance with legal and ethical standards in research and experimentation.