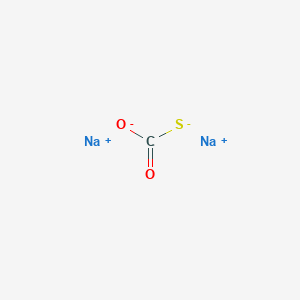
Sodium thiocarbonate
- Click on QUICK INQUIRY to receive a quote from our team of experts.
- With the quality product at a COMPETITIVE price, you can focus more on your research.
Overview
Description
Sodium thiocarbonate refers to a class of sulfur-containing salts derived from thiocarbonic acid (H₂CO₂S⁻ or HCS₃⁻). Its exact composition varies depending on the substitution of oxygen atoms with sulfur in the carbonate anion (CO₃²⁻). Common forms include monothiocarbonate (C(O)SO⁻), dithiocarbonate (C(S)SO⁻), and trithiocarbonate (CS₃²⁻). Sodium trithiocarbonate (Na₂CS₃) is particularly notable in industrial applications, such as heavy metal precipitation, where it is marketed as TMT-55 (a trisodium salt nonahydrate, Na₃C₃N₃S₃·9H₂O) . This compound effectively binds divalent metals like Cd²⁺, Cu²⁺, and Hg²⁺ via thiolate coordination, forming insoluble complexes . However, this compound derivatives are sensitive to oxidation and moisture, requiring inert synthesis conditions (e.g., solvothermal methods in evacuated ampoules) to stabilize the CS₃²⁻ anion .
Preparation Methods
Solvent-Nonsolvent Method for Controlled Precipitation
The solvent-nonsolvent method, detailed in US Patent 2893835A , avoids aqueous media to prevent decomposition and hygroscopicity issues . This approach uses a hybrid solvent system comprising:
-
3–30% polar solvent (e.g., ethanol, methanol, dimethylformamide) to dissolve reactants.
-
70–97% nonpolar diluent (e.g., hexane, benzene) to precipitate the product, ensuring easy isolation.
Reaction Mechanism
The synthesis involves the direct reaction of carbon disulfide (CS₂) with sodium sulfide (Na₂S) under anhydrous conditions:
2 + \text{Na}2\text{S} \rightarrow \text{Na}2\text{CS}3CS2+Na2S→Na2CS3
Key parameters :
-
Molar ratio : 1:1 CS₂:Na₂S, with a slight excess of CS₂ to drive completion .
-
Temperature : Room temperature (20–25°C), leveraging exothermic heat; optional gentle heating to 40°C accelerates the reaction .
-
Solvent system : 10% ethanol in hexane optimizes reactant solubility while ensuring product precipitation .
Performance Data
Example : Stirring 50 g Na₂S with 40 mL CS₂ in 10% ethanol/hexane for 2 hours yielded 44.7 g Na₂CS₃ with 0.68% residual carbon .
Aqueous Solution Method for Direct Synthesis
CN Patent 109574893A outlines an aqueous-phase synthesis for in-situ applications, where Na₂CS₃ is used immediately without isolation . This method prioritizes cost efficiency and scalability.
Reaction Protocol
-
Mixing : Carbon disulfide and 35% sodium sulfide solution are combined in a reactor.
-
Heating : Reflux at 40–45°C for 2–3 hours.
-
Concentration : The resulting solution contains 45–50% Na₂CS₃ .
Critical adjustments :
Challenges and Mitigations
Challenge | Mitigation Strategy |
---|---|
Thermal decomposition | Immediate use in downstream reactions |
Hydrogen sulfide release | Condenser recycling of CS₂ |
Example : Reacting 600 kg Na₂S (35% solution) with 200 kg CS₂ produced 3,000 L of 45–50% Na₂CS₃ solution .
Comparative Analysis of Synthesis Methods
Table 1: Method Comparison
Optimization Strategies for Industrial Scalability
Solvent Selection
-
Ethanol-hexane systems reduce costs by minimizing polar solvent use .
-
Dioxane-cyclohexane mixtures offer alternatives for temperature-sensitive reactions .
Stoichiometric Precision
Energy Efficiency
-
Exothermic heat utilization reduces external heating needs .
-
Condenser recycling of CS₂ cuts raw material costs by 15–20% .
Challenges in this compound Synthesis
Hygroscopicity
Na₂CS₃ rapidly absorbs moisture, necessitating:
Thermal Decomposition
Decomposition above 60°C limits drying methods:
Chemical Reactions Analysis
Types of Reactions
Disodium sulfidoformate undergoes various chemical reactions, including:
Oxidation: It can be oxidized to form sodium sulfate.
Reduction: It can be reduced to form sodium sulfide.
Substitution: It can undergo substitution reactions with halogens to form corresponding halides.
Common Reagents and Conditions
Oxidation: Common oxidizing agents include hydrogen peroxide and potassium permanganate.
Reduction: Reducing agents such as hydrogen gas or sodium borohydride are used.
Substitution: Halogens like chlorine or bromine are used under controlled conditions.
Major Products Formed
Oxidation: Sodium sulfate (Na₂SO₄)
Reduction: Sodium sulfide (Na₂S)
Substitution: Sodium halides (NaX, where X is a halogen)
Scientific Research Applications
Disodium sulfidoformate has several scientific research applications:
Chemistry: It is used as a reagent in various chemical syntheses and reactions.
Biology: It is used in studies involving sulfur metabolism and enzymatic reactions.
Industry: It is used in the production of rubber chemicals, sulfur dyes, and oil recovery processes.
Mechanism of Action
The mechanism of action of disodium sulfidoformate involves its ability to donate sulfur atoms in various chemical reactions. It can interact with molecular targets such as enzymes and proteins, affecting their function and activity. The pathways involved include sulfur metabolism and redox reactions.
Comparison with Similar Compounds
Structural and Spectroscopic Properties
Thiocarbonates exhibit distinct structural features compared to oxygenated carbonates and other sulfur analogs. Key differences are evident in spectroscopic
Compound | ¹³C NMR Shift (ppm) | IR Absorption (cm⁻¹) | Key Structural Feature |
---|---|---|---|
Sodium monothiocarbonate | ~165 (C(O)SO) | 183 (C=S stretch) | Regioregular TH linkages in polymers |
Sodium trithiocarbonate | 186.4 (CS₃²⁻) | 680–720 (C–S symmetric) | Trigonal-planar CS₃²⁻ anion |
Sodium carbonate (Na₂CO₃) | 167–170 (CO₃²⁻) | 880 (C–O asymmetric) | Planar CO₃²⁻ anion |
Sodium thionocarbonate | ~159 (C(S)O) | 620–650 (C=S) | Mixed C=S and C–O bonding |
- NMR Insights: Sodium monothiocarbonate polymers (e.g., poly(3) from cyclic thiocarbonate monomers) show a single ¹³C NMR peak at δ ≈ 165 ppm, indicative of uniform C(O)SO linkages. In contrast, polythionocarbonates exhibit multiple peaks (δ ≈ 159 ppm) due to TT, TH, and HH configurations .
- IR/Raman Data : Sodium trithiocarbonate’s IR spectrum shows strong C–S symmetric stretching at 680–720 cm⁻¹, distinct from carbonate’s C–O modes .
Reactivity and Thermodynamics
- Acidity: Monothiocarbonic acid (H₂CO₂S) is significantly more acidic (pKa₁ = 3.2) than carbonic acid (pKa₁ = 6.35), enhancing its reactivity in aqueous systems .
- Thermal Stability : Sodium thiocarbonate derivatives are less stable than carbonates. For example, S-alkyl thiocarbonates (e.g., SA-PeroxyTCN) are 15.7 kcal/mol more stable than O-alkyl isomers but decompose via COS extrusion under mild conditions .
- Polymerization: Cyclic thiocarbonate monomers undergo regioselective ring-opening polymerization (ROP) via acyl–sulfur cleavage, yielding polymers with strictly alternating C(O)SO linkages. This contrasts with xanthates or thionocarbonates, which form irregular backbones .
Q & A
Basic Research Questions
Q. What are the standard methodologies for synthesizing sodium thiocarbonate and confirming its purity in laboratory settings?
- Methodology :
Synthesis : this compound is typically synthesized via the reaction of carbon disulfide (CS₂) with sodium hydroxide (NaOH) under controlled conditions. For example, bubbling CS₂ through a cooled NaOH solution (e.g., 10% w/v) at 0–5°C yields Na₂CS₃ .
Purification : Recrystallization from ethanol or aqueous ethanol mixtures removes impurities.
Purity Confirmation :
- Elemental Analysis : Quantify C, S, and Na content to verify stoichiometry.
- Spectroscopic Characterization : Use IR spectroscopy to identify ν(C=S) and ν(S-S) stretches (e.g., IR absorption bands at ~985 cm⁻¹ and ~470 cm⁻¹) and Raman spectroscopy for symmetric stretching modes (~640 cm⁻¹) .
- Thermogravimetric Analysis (TGA) : Assess thermal stability and decomposition patterns .
Q. How can researchers distinguish this compound from structurally similar sulfur-containing compounds (e.g., thiocarbonates vs. dithiocarbonates)?
- Methodology :
Spectroscopic Differentiation :
- IR/Raman : Thiocarbonates (CS₃²⁻) exhibit distinct ν(C-S) and ν(S-S) vibrations compared to dithiocarbonates (CS₂O²⁻) or trithiocarbonates (CS₃²⁻). For example, Na₂CS₃ shows Raman bands at ~640 cm⁻¹, absent in dithiocarbonates .
X-ray Diffraction (XRD) : Compare crystallographic data with reference structures from databases like the Cambridge Structural Database.
Reactivity Tests : this compound reacts with acids to release CS₂ gas, whereas dithiocarbonates may produce CO₂ or other byproducts .
Q. What are the critical considerations for handling and storing this compound in experimental workflows?
- Methodology :
Moisture Sensitivity : Store in airtight containers under inert gas (e.g., N₂) to prevent hydrolysis, which can degrade CS₃²⁻ to carbonate and sulfide species .
Temperature Control : Avoid prolonged exposure to temperatures >40°C, as decomposition accelerates.
Safety Protocols : Use fume hoods for synthesis and handling due to CS₂ volatility, and wear PPE to mitigate skin/eye contact risks .
Advanced Research Questions
Q. How can computational methods (e.g., DFT calculations) resolve discrepancies in experimental spectroscopic data for this compound derivatives?
- Methodology :
DFT Modeling : Optimize molecular geometries using software like Gaussian or ORCA to simulate IR/Raman spectra. Compare computed vibrational frequencies (e.g., ~985 cm⁻¹ for C=S) with experimental data to validate assignments .
Error Analysis : Quantify deviations between experimental and theoretical results (e.g., scaling factors for vibrational modes) to refine computational parameters.
Cross-Validation : Use multiple spectroscopic techniques (e.g., NMR for solution-phase behavior) to reconcile structural ambiguities .
Q. What experimental design strategies optimize reaction conditions for this compound in complex synthetic pathways (e.g., polymer ROP or cross-coupling reactions)?
- Methodology :
Design of Experiments (DoE) : Apply factorial designs to evaluate variables (e.g., temperature, solvent polarity, catalyst loading) on reaction efficiency. For example, in ring-opening polymerization (ROP) of cyclic thiocarbonates, DBU catalyst concentration significantly impacts polymerization rate .
Kinetic Studies : Use in-situ monitoring (e.g., FTIR or Raman spectroscopy) to track reaction progress and identify rate-limiting steps.
Post-Reaction Analysis : Isolate intermediates via column chromatography and characterize by mass spectrometry to elucidate mechanistic pathways .
Q. How can researchers address reproducibility challenges in this compound-based studies, particularly when replicating historical syntheses (e.g., pre-1950 methods)?
- Methodology :
Literature Critical Analysis : Cross-reference historical procedures (e.g., Marion Jeanette Sears’ 1936 thesis) with modern safety and analytical standards .
Controlled Replication : Systematically vary parameters (e.g., reagent purity, stirring rates) to identify undocumented variables affecting yield.
Archival Data Integration : Compare historical spectroscopic data (if available) with contemporary instrumentation outputs to calibrate measurements .
Q. Methodological Notes
- Hypothesis Testing : Frame research questions using PICOT criteria (Population, Intervention, Comparison, Outcome, Time) to ensure clarity and testability .
- Data Reporting : Adhere to journal guidelines (e.g., Beilstein Journal of Organic Chemistry) for experimental reproducibility, including detailed synthesis protocols and raw spectral data in supplementary materials .
- Ethical Compliance : Document safety protocols for CS₂ handling, referencing SDS guidelines (e.g., GHS hazard codes) .
Properties
Molecular Formula |
CNa2O2S |
---|---|
Molecular Weight |
122.06 g/mol |
IUPAC Name |
disodium;sulfidoformate |
InChI |
InChI=1S/CH2O2S.2Na/c2-1(3)4;;/h4H,(H,2,3);;/q;2*+1/p-2 |
InChI Key |
VKJSPOIGADJDPH-UHFFFAOYSA-L |
Canonical SMILES |
C(=O)([O-])[S-].[Na+].[Na+] |
Origin of Product |
United States |
Disclaimer and Information on In-Vitro Research Products
Please be aware that all articles and product information presented on BenchChem are intended solely for informational purposes. The products available for purchase on BenchChem are specifically designed for in-vitro studies, which are conducted outside of living organisms. In-vitro studies, derived from the Latin term "in glass," involve experiments performed in controlled laboratory settings using cells or tissues. It is important to note that these products are not categorized as medicines or drugs, and they have not received approval from the FDA for the prevention, treatment, or cure of any medical condition, ailment, or disease. We must emphasize that any form of bodily introduction of these products into humans or animals is strictly prohibited by law. It is essential to adhere to these guidelines to ensure compliance with legal and ethical standards in research and experimentation.