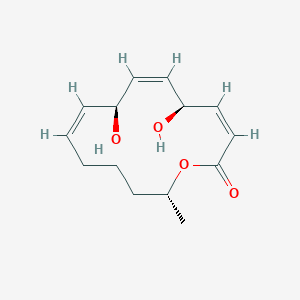
Mutolide
Overview
Description
Mutolide is a 14-membered macrolide compound discovered through chemical screening of the culture broth of the fungus F-24′707y, which was obtained after ultraviolet mutagenesis of the wild type strain. This strain typically produces the spirobisnaphthalene cladospirone bisepoxide . This compound has garnered interest due to its unique structure and potential biological activities.
Preparation Methods
Synthetic Routes and Reaction Conditions
The preparation of mutolide involves the use of acetate and malonate as precursors. The biogenetic origin of the carbon skeleton and hydroxy groups was verified by feeding sodium [1-13C]acetate and 18O2 to growing cultures of the fungus . The structure of this compound was established through detailed spectroscopic analysis, X-ray analysis, and derivatization .
Industrial Production Methods
Industrial production methods for this compound are not extensively documented. the use of ultraviolet mutagenesis to induce the production of this compound in fungal cultures suggests that similar biotechnological approaches could be employed for large-scale production.
Chemical Reactions Analysis
Types of Reactions
Mutolide undergoes various chemical reactions, including oxidation and reduction. The compound’s structure allows it to participate in these reactions, which can lead to the formation of different derivatives.
Common Reagents and Conditions
Common reagents used in the reactions involving this compound include oxidizing agents and reducing agents. The specific conditions for these reactions depend on the desired outcome and the nature of the derivatives being synthesized.
Major Products Formed
The major products formed from the reactions of this compound include various oxidized and reduced derivatives
Scientific Research Applications
Mutolide has shown significant potential in scientific research, particularly in the fields of chemistry, biology, and medicine. Some of its notable applications include:
Anti-inflammatory Properties: This compound has been found to mitigate lipopolysaccharide-induced secretion of pro-inflammatory cytokines such as tumor necrosis factor-alpha and interleukin-6 from THP-1 cells and human peripheral blood mononuclear cells. This suggests its potential use in treating inflammatory diseases.
Cytotoxic Activity: This compound has displayed significant cytotoxic activity against human colon cancer cells, with an IC50 value of approximately 12 micromolar. This indicates its potential as an anticancer agent.
Inhibition of CFTR-mediated Chloride Secretion: This compound has shown a potent inhibitory effect on cystic fibrosis transmembrane conductance regulator-mediated chloride secretion in human intestinal epithelial cells.
Mechanism of Action
Mutolide exerts its effects primarily through the inhibition of nuclear factor kappa B activation and translocation from the cytoplasm into the nucleus . This inhibition prevents the transcription of pro-inflammatory cytokines, thereby reducing inflammation. Additionally, this compound does not significantly affect the activity of p38 mitogen-activated protein kinase, a serine/threonine kinase involved in cell cycle proliferation and cytokine secretion .
Comparison with Similar Compounds
Mutolide is unique due to its specific structure and biological activities. Similar compounds include other macrolides such as:
Nigrosporolide: Another 14-membered macrolide with similar structural features.
These compounds share some structural similarities with this compound but differ in their specific biological activities and applications.
Properties
IUPAC Name |
(3Z,5S,6Z,8S,9Z,14R)-5,8-dihydroxy-14-methyl-1-oxacyclotetradeca-3,6,9-trien-2-one | |
---|---|---|
Details | Computed by LexiChem 2.6.6 (PubChem release 2019.06.18) | |
Source | PubChem | |
URL | https://pubchem.ncbi.nlm.nih.gov | |
Description | Data deposited in or computed by PubChem | |
InChI |
InChI=1S/C14H20O4/c1-11-5-3-2-4-6-12(15)7-8-13(16)9-10-14(17)18-11/h4,6-13,15-16H,2-3,5H2,1H3/b6-4-,8-7-,10-9-/t11-,12+,13+/m1/s1 | |
Details | Computed by InChI 1.0.5 (PubChem release 2019.06.18) | |
Source | PubChem | |
URL | https://pubchem.ncbi.nlm.nih.gov | |
Description | Data deposited in or computed by PubChem | |
InChI Key |
SAORJUUJHIHDBZ-GLJCHZBLSA-N | |
Details | Computed by InChI 1.0.5 (PubChem release 2019.06.18) | |
Source | PubChem | |
URL | https://pubchem.ncbi.nlm.nih.gov | |
Description | Data deposited in or computed by PubChem | |
Canonical SMILES |
CC1CCCC=CC(C=CC(C=CC(=O)O1)O)O | |
Details | Computed by OEChem 2.1.5 (PubChem release 2019.06.18) | |
Source | PubChem | |
URL | https://pubchem.ncbi.nlm.nih.gov | |
Description | Data deposited in or computed by PubChem | |
Isomeric SMILES |
C[C@@H]1CCC/C=C\[C@@H](/C=C\[C@@H](/C=C\C(=O)O1)O)O | |
Details | Computed by OEChem 2.1.5 (PubChem release 2019.06.18) | |
Source | PubChem | |
URL | https://pubchem.ncbi.nlm.nih.gov | |
Description | Data deposited in or computed by PubChem | |
Molecular Formula |
C14H20O4 | |
Details | Computed by PubChem 2.1 (PubChem release 2019.06.18) | |
Source | PubChem | |
URL | https://pubchem.ncbi.nlm.nih.gov | |
Description | Data deposited in or computed by PubChem | |
Molecular Weight |
252.31 g/mol | |
Details | Computed by PubChem 2.1 (PubChem release 2021.05.07) | |
Source | PubChem | |
URL | https://pubchem.ncbi.nlm.nih.gov | |
Description | Data deposited in or computed by PubChem | |
Retrosynthesis Analysis
AI-Powered Synthesis Planning: Our tool employs the Template_relevance Pistachio, Template_relevance Bkms_metabolic, Template_relevance Pistachio_ringbreaker, Template_relevance Reaxys, Template_relevance Reaxys_biocatalysis model, leveraging a vast database of chemical reactions to predict feasible synthetic routes.
One-Step Synthesis Focus: Specifically designed for one-step synthesis, it provides concise and direct routes for your target compounds, streamlining the synthesis process.
Accurate Predictions: Utilizing the extensive PISTACHIO, BKMS_METABOLIC, PISTACHIO_RINGBREAKER, REAXYS, REAXYS_BIOCATALYSIS database, our tool offers high-accuracy predictions, reflecting the latest in chemical research and data.
Strategy Settings
Precursor scoring | Relevance Heuristic |
---|---|
Min. plausibility | 0.01 |
Model | Template_relevance |
Template Set | Pistachio/Bkms_metabolic/Pistachio_ringbreaker/Reaxys/Reaxys_biocatalysis |
Top-N result to add to graph | 6 |
Feasible Synthetic Routes
Disclaimer and Information on In-Vitro Research Products
Please be aware that all articles and product information presented on BenchChem are intended solely for informational purposes. The products available for purchase on BenchChem are specifically designed for in-vitro studies, which are conducted outside of living organisms. In-vitro studies, derived from the Latin term "in glass," involve experiments performed in controlled laboratory settings using cells or tissues. It is important to note that these products are not categorized as medicines or drugs, and they have not received approval from the FDA for the prevention, treatment, or cure of any medical condition, ailment, or disease. We must emphasize that any form of bodily introduction of these products into humans or animals is strictly prohibited by law. It is essential to adhere to these guidelines to ensure compliance with legal and ethical standards in research and experimentation.