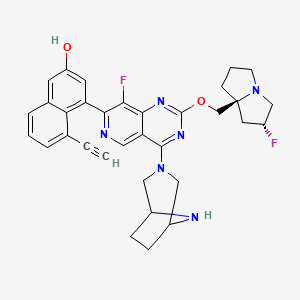
KRAS G12D inhibitor 1
Overview
Description
KRAS G12D inhibitor 1 (CAS: N/A) is a potent small-molecule inhibitor targeting the KRAS G12D mutation, a prevalent oncogenic driver in pancreatic, colorectal, and lung cancers. According to MedChemExpress (MCE), this compound exhibits an IC50 of 0.8 nM against KRAS G12D-mediated ERK phosphorylation, demonstrating high selectivity and efficacy in preclinical models . Its mechanism involves stabilizing the inactive GDP-bound conformation of KRAS G12D, thereby blocking downstream MAPK/ERK signaling. While structural details remain proprietary, its pharmacological profile positions it as a promising candidate for further development.
Preparation Methods
Synthetic Routes and Reaction Conditions
The synthesis of KRAS G12D inhibitor 1 involves a series of chemical reactions, including the formation of a salt bridge with the Asp12 residue of the KRAS protein. The synthetic route typically starts with the preparation of a piperazine moiety, which is then coupled with other chemical intermediates to form the final inhibitor .
Industrial Production Methods
Industrial production of this compound involves large-scale synthesis using optimized reaction conditions to ensure high yield and purity. The process includes steps such as crystallization, purification, and quality control to meet pharmaceutical standards .
Chemical Reactions Analysis
Types of Reactions
KRAS G12D inhibitor 1 undergoes various chemical reactions, including:
Oxidation: Involves the addition of oxygen or the removal of hydrogen.
Reduction: Involves the addition of hydrogen or the removal of oxygen.
Substitution: Involves the replacement of one functional group with another
Common Reagents and Conditions
Common reagents used in these reactions include oxidizing agents like hydrogen peroxide, reducing agents like sodium borohydride, and various catalysts to facilitate the reactions. The conditions often involve controlled temperatures and pH levels to ensure the desired reaction outcomes .
Major Products Formed
The major products formed from these reactions include various intermediates that are further processed to yield the final this compound. These intermediates are characterized by their specific chemical structures and properties .
Scientific Research Applications
KRAS G12D inhibitor 1 has a wide range of scientific research applications, including:
Chemistry: Used as a model compound to study the chemical properties and reactivity of KRAS inhibitors.
Biology: Employed in cellular studies to understand the role of KRAS mutations in cell signaling and proliferation.
Medicine: Investigated as a potential therapeutic agent for treating cancers with KRAS G12D mutations, particularly pancreatic cancer
Industry: Utilized in the development of new drugs and therapeutic strategies targeting KRAS mutations
Mechanism of Action
KRAS G12D inhibitor 1 exerts its effects by binding to the KRAS protein at the G12D mutation site. This binding disrupts the interaction between KRAS and its downstream effectors, such as the RAF-MEK-ERK signaling pathway. By inhibiting this pathway, the compound effectively reduces cell proliferation and induces apoptosis in cancer cells .
Comparison with Similar Compounds
Comparative Analysis with Similar KRAS G12D Inhibitors
TH-Z835 (Salt Bridge-Forming Inhibitor)
Mechanism : TH-Z835, developed by Mao et al., forms a salt bridge with Asp12 in both GDP- and GTP-bound KRAS G12D, inducing a conformational shift in the switch II region. This stabilizes a cryptic ligand-binding pocket beneath switch II, as revealed by co-crystal structures (PDB: 7EWA, 7EWB) .
Efficacy :
- Binds KRAS G12D with comparable affinity in GDP/GTP states (Kd ~100–200 nM).
- Reduces tumor growth in xenograft models and synergizes with MEK inhibitors . Limitations: Potential off-target effects on Rho, Ran, Arf, and Rab GTPases due to structural similarities in nucleotide-binding domains .
MRTX1133 (Non-Covalent Inhibitor)
Mechanism : MRTX1133 selectively binds the switch II pocket (S-IIP) of KRAS G12D using a piperazinyl group to form ionic interactions with Asp12. It targets both active (GTP-bound) and inactive (GDP-bound) states .
Efficacy :
- Achieves tumor regression in pancreatic ductal adenocarcinoma (PDAC) murine models.
- Reduces phosphorylated ERK (pERK) by >80% at 100 nM .
Clinical Status : Phase I/II trials ongoing for PDAC and colorectal cancer .
RMC-9805 (Covalent Tri-Complex Inhibitor)
Mechanism: Utilizes cyclophilin A as a chaperone to form a non-covalent binary complex, which then binds KRAS G12D-GTP to create a tri-complex. This irreversibly blocks effector interactions . Efficacy:
- Shows >90% inhibition of KRAS signaling in preclinical PDAC models.
- Minimal wild-type KRAS inhibition, reducing toxicity risks .
Clinical Status : Phase I trials initiated in 2023 .
PU1-1 (Purine/Pyrimidine-Based Inhibitor)
Mechanism : PU1-1 reduces KRAS-GTP levels and downregulates pERK via undefined allosteric interactions, likely targeting nucleotide exchange .
Efficacy :
- IC50 of 1.2 µM in antiproliferative assays.
- Induces apoptosis in KRAS G12D-mutant cell lines .
Limitations : Lower potency compared to covalent inhibitors; requires structural optimization .
BI-2852 and Computational Hits (Pharmacophore-Based Inhibitors)
Mechanism : BI-2852, identified via chemical screening, blocks GEF/GAP/effector interactions. Virtual screening using a pharmacophore model (two HBD, one HBA, two aromatic rings) identified Hit1 and Hit2 with higher binding free energy than BI-2852 .
Efficacy :
- Hit1 and Hit2 exhibit Kd values of ~50 nM in silico.
- PCA analysis confirms stable interactions with KRAS G12D . Limitations: Limited experimental validation; structural diversity in training sets may bias pharmacophore models .
TUS-007 (Proteasome-Mediated Degrader)
Mechanism : A proteolysis-targeting chimera (PROTAC) that recruits the 26S proteasome to degrade KRAS G12D. Modified from a proteasome inhibitor, it avoids proteasome inhibition .
Efficacy :
- DC50 of 4 µM in vitro.
- Reduces KRAS G12D/V levels by >70% in biochemical assays .
Limitations : Lower cellular potency compared to direct inhibitors.
HBW-012336 (Oral Inhibitor with Tissue Enrichment)
Mechanism : An oral KRAS G12D inhibitor with enhanced bioavailability and tissue-specific enrichment in the pancreas, intestines, and lungs .
Efficacy :
Comparative Data Table
Key Research Findings and Challenges
- Structural Insights : Co-crystal structures of TH-Z835 and MRTX1133 reveal conserved interactions with Asp12 and switch II, informing iterative drug design .
- Clinical Progress : MRTX1133 and RMC-9805 lead clinical development, with early trials focusing on PDAC and colorectal cancers .
- Unmet Needs : Improving oral bioavailability (e.g., HBW-012336) and mitigating off-target effects remain critical hurdles .
Biological Activity
The KRAS G12D mutation is a pivotal driver in various cancers, particularly pancreatic ductal adenocarcinoma (PDAC), where it is found in approximately 37% of cases. The development of targeted therapies against this mutation has garnered significant attention, with MRTX1133 emerging as a promising non-covalent inhibitor. This article explores the biological activity of MRTX1133, detailing its mechanisms, efficacy in preclinical models, and implications for future cancer therapies.
MRTX1133 selectively inhibits the KRAS G12D mutant protein by binding to its active site, thereby blocking its interaction with downstream effectors such as CRAF and inhibiting the MAPK signaling pathway. This inhibition is crucial because KRAS G12D mutations lead to uncontrolled cell proliferation and survival pathways that contribute to tumorigenesis. The compound's binding affinity has been characterized with an IC50 value as low as 6.1 nM in various KRAS G12D mutant cell lines, demonstrating its potency against this oncogenic variant .
Preclinical Efficacy
In Vitro Studies:
MRTX1133 has shown remarkable efficacy in vitro across multiple cancer cell lines harboring the KRAS G12D mutation. For instance, studies report that treatment with MRTX1133 leads to significant reductions in cell viability and downstream signaling activity, evidenced by decreased levels of phosphorylated ERK1/2 .
In Vivo Studies:
In mouse models of human pancreatic cancer, MRTX1133 not only halted tumor growth but also induced substantial tumor regressions. In one study involving autochthonous PDAC models with intact immune systems, MRTX1133 treatment resulted in complete or near-complete remissions within 14 days . Importantly, the presence of CD8+ T cells was essential for the full antitumor effect of MRTX1133, indicating that the drug may enhance anti-tumor immunity .
Impact on Tumor Microenvironment
MRTX1133 treatment has been shown to reprogram the tumor microenvironment (TME). It increases the infiltration of CD8+ T cells while decreasing myeloid cell infiltration and altering cancer-associated fibroblasts . This shift not only enhances the immune response against tumors but also suggests potential for combination therapies with immune checkpoint inhibitors (ICBs), which could further augment therapeutic efficacy .
Case Studies and Clinical Implications
Recent clinical trials have begun evaluating MRTX1133's safety and efficacy in humans. Preliminary results indicate that patients with advanced PDAC exhibit promising responses to this targeted therapy, with significant tumor shrinkage observed in several cases . Additionally, the drug's ability to overcome some resistance mechanisms associated with KRAS mutations underscores its potential as a cornerstone therapy for KRAS-driven malignancies.
Data Summary
Study | Model | IC50 (nM) | Efficacy | Notes |
---|---|---|---|---|
Salem et al. (2022) | PDAC cell lines | 6.1 | Significant reduction in viability | Selective for KRAS G12D |
NCI Study (2023) | Mouse models | - | Tumor shrinkage observed | Required CD8+ T cells for full effect |
PubMed Study (2023) | Patient-derived organoids | - | Regression of advanced PDAC | Synergistic potential with ICB |
Q & A
Basic Research Questions
Q. What is the molecular mechanism by which KRAS G12D inhibitor 1 selectively targets the G12D mutation?
this compound binds to the active GTP-bound state of KRAS G12D, disrupting its interaction with downstream effectors like RAF1. Structural studies reveal that the inhibitor forms a salt bridge with the aspartic acid residue at position 12 (mutated from glycine), a key feature absent in wild-type KRAS. This interaction stabilizes the inactive conformation of KRAS, inhibiting ERK phosphorylation (IC50 = 0.8 nM) .
Q. Which in vitro assays are most reliable for evaluating KRAS G12D inhibitor efficacy?
- CellTiter-Glo Assays : Measure proliferation inhibition in KRAS G12D-mutant cell lines (e.g., pancreatic PANC-1 or colorectal SW1463 cells) .
- Nucleotide Exchange Assays : Use BODIPY®-GDP to monitor GTP/GDP binding dynamics, with protocols allowing titration of inhibitors at fixed GTP concentrations .
- Phospho-ERK (p-ERK) ELISAs : Quantify downstream MAPK pathway inhibition, validated by sub-nanomolar IC50 values in cellular models .
Q. How do structural studies inform the design of KRAS G12D inhibitors?
X-ray crystallography (e.g., PDB: 7EW9) and isothermal titration calorimetry (ITC) reveal critical binding interactions. For example, inhibitors like TH-Z827 exploit the shallow pocket near residue 12, forming hydrogen bonds with Q61 and hydrophobic interactions with H94. These insights guide optimization of binding affinity and selectivity .
Advanced Research Questions
Q. What experimental strategies address resistance mechanisms in KRAS G12D inhibitor-treated tumors?
Resistance often arises from adaptive feedback loops (e.g., RTK/MAPK reactivation). Preclinical models combine KRAS G12D inhibitors with MEK inhibitors (e.g., avutometinib) to block compensatory pathways. In vivo studies in pancreatic ductal adenocarcinoma (PDAC) CDX models demonstrate synergistic tumor regression (e.g., 30 mg/kg twice daily dosing) .
Q. How can computational methods enhance KRAS G12D inhibitor discovery?
- Pharmacophore Modeling : Identifies essential chemical features (e.g., two hydrogen bond donors, aromatic rings) for KRAS G12D inhibition .
- Molecular Dynamics (MD) Simulations : Assess binding stability over 100-ns trajectories, highlighting interactions with residues Y96 and D119 .
- Virtual Screening : Libraries like ZINC or InterBioScreen (>214,000 compounds) are filtered using Glide docking (Schrödinger Suite) to prioritize candidates with sub-μM affinity .
Q. What in vivo models best recapitulate KRAS G12D-driven tumor biology for preclinical testing?
- Cell-Derived Xenografts (CDX) : Use KRAS G12D-mutant PDAC or CRC cell lines (e.g., MIA PaCa-2) to evaluate tumor growth inhibition and pharmacodynamics (e.g., p-ERK suppression) .
- Intracranial Models : Assess blood-brain barrier penetration in glioblastoma models, with efficacy demonstrated at 10 mg/kg BID dosing .
Q. Methodological Considerations
Q. How should researchers validate target engagement in KRAS G12D inhibition studies?
- ITC Assays : Measure binding thermodynamics (KD values) using purified KRAS G12D protein and inhibitors in buffer containing 5 mM MgCl2 and 1 mM TCEP .
- KRAS-CRAF Interaction Assays : Luminescence-based assays quantify disruption of KRAS-RAF1 binding in HEK 293T cells transfected with KRAS G12D-CRAF plasmids .
Q. What are key pharmacokinetic parameters to optimize for oral KRAS G12D inhibitors?
- Bioavailability : Structural modifications (e.g., HBW-012336) improve absorption by reducing efflux via P-glycoprotein .
- Tissue Enrichment : Compounds like SHR1127 show preferential accumulation in pancreas and lungs, critical for targeting PDAC and NSCLC .
Q. Notes
Properties
IUPAC Name |
4-[4-(3,8-diazabicyclo[3.2.1]octan-3-yl)-8-fluoro-2-[[(2R,8S)-2-fluoro-1,2,3,5,6,7-hexahydropyrrolizin-8-yl]methoxy]pyrido[4,3-d]pyrimidin-7-yl]-5-ethynylnaphthalen-2-ol | |
---|---|---|
Details | Computed by Lexichem TK 2.7.0 (PubChem release 2021.08.13) | |
Source | PubChem | |
URL | https://pubchem.ncbi.nlm.nih.gov | |
Description | Data deposited in or computed by PubChem | |
InChI |
InChI=1S/C33H32F2N6O2/c1-2-19-5-3-6-20-11-24(42)12-25(27(19)20)29-28(35)30-26(14-36-29)31(40-16-22-7-8-23(17-40)37-22)39-32(38-30)43-18-33-9-4-10-41(33)15-21(34)13-33/h1,3,5-6,11-12,14,21-23,37,42H,4,7-10,13,15-18H2/t21-,22?,23?,33+/m1/s1 | |
Details | Computed by InChI 1.0.6 (PubChem release 2021.08.13) | |
Source | PubChem | |
URL | https://pubchem.ncbi.nlm.nih.gov | |
Description | Data deposited in or computed by PubChem | |
InChI Key |
HQVNRYKTWPMSGZ-ADTQRWQWSA-N | |
Details | Computed by InChI 1.0.6 (PubChem release 2021.08.13) | |
Source | PubChem | |
URL | https://pubchem.ncbi.nlm.nih.gov | |
Description | Data deposited in or computed by PubChem | |
Canonical SMILES |
C#CC1=CC=CC2=CC(=CC(=C21)C3=NC=C4C(=C3F)N=C(N=C4N5CC6CCC(C5)N6)OCC78CCCN7CC(C8)F)O | |
Details | Computed by OEChem 2.3.0 (PubChem release 2021.08.13) | |
Source | PubChem | |
URL | https://pubchem.ncbi.nlm.nih.gov | |
Description | Data deposited in or computed by PubChem | |
Isomeric SMILES |
C#CC1=CC=CC2=CC(=CC(=C21)C3=NC=C4C(=C3F)N=C(N=C4N5CC6CCC(C5)N6)OC[C@@]78CCCN7C[C@@H](C8)F)O | |
Details | Computed by OEChem 2.3.0 (PubChem release 2021.08.13) | |
Source | PubChem | |
URL | https://pubchem.ncbi.nlm.nih.gov | |
Description | Data deposited in or computed by PubChem | |
Molecular Formula |
C33H32F2N6O2 | |
Details | Computed by PubChem 2.1 (PubChem release 2021.08.13) | |
Source | PubChem | |
URL | https://pubchem.ncbi.nlm.nih.gov | |
Description | Data deposited in or computed by PubChem | |
Molecular Weight |
582.6 g/mol | |
Details | Computed by PubChem 2.1 (PubChem release 2021.08.13) | |
Source | PubChem | |
URL | https://pubchem.ncbi.nlm.nih.gov | |
Description | Data deposited in or computed by PubChem | |
Retrosynthesis Analysis
AI-Powered Synthesis Planning: Our tool employs the Template_relevance Pistachio, Template_relevance Bkms_metabolic, Template_relevance Pistachio_ringbreaker, Template_relevance Reaxys, Template_relevance Reaxys_biocatalysis model, leveraging a vast database of chemical reactions to predict feasible synthetic routes.
One-Step Synthesis Focus: Specifically designed for one-step synthesis, it provides concise and direct routes for your target compounds, streamlining the synthesis process.
Accurate Predictions: Utilizing the extensive PISTACHIO, BKMS_METABOLIC, PISTACHIO_RINGBREAKER, REAXYS, REAXYS_BIOCATALYSIS database, our tool offers high-accuracy predictions, reflecting the latest in chemical research and data.
Strategy Settings
Precursor scoring | Relevance Heuristic |
---|---|
Min. plausibility | 0.01 |
Model | Template_relevance |
Template Set | Pistachio/Bkms_metabolic/Pistachio_ringbreaker/Reaxys/Reaxys_biocatalysis |
Top-N result to add to graph | 6 |
Feasible Synthetic Routes
Disclaimer and Information on In-Vitro Research Products
Please be aware that all articles and product information presented on BenchChem are intended solely for informational purposes. The products available for purchase on BenchChem are specifically designed for in-vitro studies, which are conducted outside of living organisms. In-vitro studies, derived from the Latin term "in glass," involve experiments performed in controlled laboratory settings using cells or tissues. It is important to note that these products are not categorized as medicines or drugs, and they have not received approval from the FDA for the prevention, treatment, or cure of any medical condition, ailment, or disease. We must emphasize that any form of bodily introduction of these products into humans or animals is strictly prohibited by law. It is essential to adhere to these guidelines to ensure compliance with legal and ethical standards in research and experimentation.