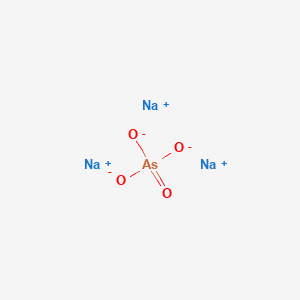
Trisodium arsenate
Overview
Description
Trisodium arsenate, with the chemical formula Na₃AsO₄, is an inorganic compound that features arsenic in its highest oxidation state. It is typically observed as a white or colorless crystalline solid and is highly toxic. This compound is often handled as the dodecahydrate form, Na₃AsO₄·12H₂O, and is known for its solubility in water .
Preparation Methods
Synthetic Routes and Reaction Conditions: Trisodium arsenate can be synthesized by neutralizing arsenic acid (H₃AsO₄) with sodium hydroxide (NaOH). The reaction is as follows: [ \text{H}_3\text{AsO}_4 + 3\text{NaOH} \rightarrow \text{Na}_3\text{AsO}_4 + 3\text{H}_2\text{O} ]
Industrial Production Methods: In industrial settings, this compound is produced by reacting arsenic trioxide (As₂O₃) with sodium carbonate (Na₂CO₃) in the presence of water. The reaction is: [ \text{As}_2\text{O}_3 + 3\text{Na}_2\text{CO}_3 + 3\text{H}_2\text{O} \rightarrow 2\text{Na}_3\text{AsO}_4 + 3\text{CO}_2 ]
Types of Reactions:
Oxidation: this compound can undergo oxidation reactions, particularly in the presence of strong oxidizing agents.
Reduction: It can be reduced to arsenite (AsO₃³⁻) under reducing conditions.
Substitution: this compound can participate in substitution reactions where the arsenate ion (AsO₄³⁻) is replaced by other anions.
Common Reagents and Conditions:
Oxidation: Strong oxidizing agents such as potassium permanganate (KMnO₄) or hydrogen peroxide (H₂O₂).
Reduction: Reducing agents like sodium borohydride (NaBH₄) or zinc in acidic conditions.
Substitution: Various anions like phosphate (PO₄³⁻) or sulfate (SO₄²⁻) under appropriate conditions.
Major Products:
Oxidation: Formation of higher oxidation state compounds.
Reduction: Formation of arsenite compounds.
Substitution: Formation of substituted arsenate compounds.
Scientific Research Applications
Trisodium arsenate has diverse applications in scientific research:
Chemistry: Used as a reagent in analytical chemistry for the detection and quantification of various elements.
Biology: Employed in studies related to enzyme inhibition and cellular metabolism.
Medicine: Investigated for its potential therapeutic effects, particularly in the treatment of certain types of cancer.
Industry: Utilized in the production of pesticides, herbicides, and wood preservatives due to its toxic properties
Mechanism of Action
The mechanism by which trisodium arsenate exerts its effects involves the inhibition of enzymes that contain sulfhydryl groups. The arsenate ion can replace phosphate in biochemical pathways, disrupting cellular processes. This leads to the inhibition of ATP production and cellular respiration, ultimately causing cell death .
Comparison with Similar Compounds
- Disodium hydrogen arsenate (Na₂HAsO₄)
- Sodium dihydrogen arsenate (NaH₂AsO₄)
- Arsenic trioxide (As₂O₃)
Comparison:
- Disodium hydrogen arsenate and sodium dihydrogen arsenate are similar in that they also contain arsenate ions but differ in the number of sodium ions and their solubility properties.
- Arsenic trioxide is a precursor in the synthesis of trisodium arsenate and has different oxidation states and applications.
This compound is unique due to its high solubility in water and its ability to form stable hydrates, making it particularly useful in various industrial and research applications .
Biological Activity
Trisodium arsenate (Na₃AsO₄) is a compound of significant interest in both environmental and health sciences due to its biological activity and potential toxicological effects. This article explores the biological activity of this compound, focusing on its mechanisms of action, effects on various biological systems, case studies, and research findings.
This compound is a pentavalent arsenic compound that acts primarily as an oxidizing agent. Its biological activity is attributed to its ability to generate reactive oxygen species (ROS) and induce oxidative stress, leading to cellular damage. The conversion of pentavalent arsenic to trivalent arsenic (more toxic) within biological systems further complicates its effects.
Key Mechanisms:
- Oxidative Stress Induction: this compound exposure leads to increased levels of malondialdehyde (MDA), a marker of lipid peroxidation, indicating oxidative damage to cellular membranes .
- Enzymatic Activity Alteration: Studies have shown that this compound affects the activity of various enzymes involved in metabolic processes, including hexokinase and lactate dehydrogenase, while inhibiting others like glucose-6-phosphatase .
- Cell Cycle Disruption: Exposure to sodium arsenate has been linked to alterations in cell cycle progression, particularly in lymphoid cells, leading to increased apoptosis .
Toxicological Effects
The toxicological profile of this compound varies based on exposure levels and duration. Acute exposure can lead to gastrointestinal distress, cardiovascular complications, and neurological symptoms.
Case Studies
- Accidental Ingestion: A retrospective review of 149 cases involving accidental ingestion of sodium arsenate-containing ant killer revealed that most patients were asymptomatic. Only a few experienced mild symptoms such as vomiting and diarrhea, which resolved without medical intervention .
- Severe Toxicity Case: A case involving a 32-year-old male who ingested 900 mg of sodium arsenate resulted in severe gastrointestinal symptoms and acute renal failure. After treatment with chelation therapy, the patient recovered fully over several months .
Research Findings
Recent studies have provided insights into the biological effects of this compound:
- Renal Impact: Research indicates that sodium arsenate induces oxidative stress in renal tissues, significantly affecting the activity of antioxidant enzymes like superoxide dismutase and catalase, leading to renal damage .
- Hematological Effects: Chronic exposure has been associated with hematological changes, including anemia and peripheral neuropathy. These effects are particularly pronounced in populations exposed to contaminated water sources .
Data Table: Summary of Biological Effects
Properties
IUPAC Name |
trisodium;arsorate | |
---|---|---|
Source | PubChem | |
URL | https://pubchem.ncbi.nlm.nih.gov | |
Description | Data deposited in or computed by PubChem | |
InChI |
InChI=1S/AsH3O4.3Na/c2-1(3,4)5;;;/h(H3,2,3,4,5);;;/q;3*+1/p-3 | |
Source | PubChem | |
URL | https://pubchem.ncbi.nlm.nih.gov | |
Description | Data deposited in or computed by PubChem | |
InChI Key |
CDBAKLRDFBGJOX-UHFFFAOYSA-K | |
Source | PubChem | |
URL | https://pubchem.ncbi.nlm.nih.gov | |
Description | Data deposited in or computed by PubChem | |
Canonical SMILES |
[O-][As](=O)([O-])[O-].[Na+].[Na+].[Na+] | |
Source | PubChem | |
URL | https://pubchem.ncbi.nlm.nih.gov | |
Description | Data deposited in or computed by PubChem | |
Molecular Formula |
AsNa3O4 | |
Source | PubChem | |
URL | https://pubchem.ncbi.nlm.nih.gov | |
Description | Data deposited in or computed by PubChem | |
Related CAS |
7631-89-2 (Parent) | |
Record name | Sodium arsenate | |
Source | ChemIDplus | |
URL | https://pubchem.ncbi.nlm.nih.gov/substance/?source=chemidplus&sourceid=0013464385 | |
Description | ChemIDplus is a free, web search system that provides access to the structure and nomenclature authority files used for the identification of chemical substances cited in National Library of Medicine (NLM) databases, including the TOXNET system. | |
DSSTOX Substance ID |
DTXSID9039367 | |
Record name | Trisodium arsenate | |
Source | EPA DSSTox | |
URL | https://comptox.epa.gov/dashboard/DTXSID9039367 | |
Description | DSSTox provides a high quality public chemistry resource for supporting improved predictive toxicology. | |
Molecular Weight |
207.889 g/mol | |
Source | PubChem | |
URL | https://pubchem.ncbi.nlm.nih.gov | |
Description | Data deposited in or computed by PubChem | |
CAS No. |
13464-38-5, 64070-83-3 | |
Record name | Sodium arsenate | |
Source | ChemIDplus | |
URL | https://pubchem.ncbi.nlm.nih.gov/substance/?source=chemidplus&sourceid=0013464385 | |
Description | ChemIDplus is a free, web search system that provides access to the structure and nomenclature authority files used for the identification of chemical substances cited in National Library of Medicine (NLM) databases, including the TOXNET system. | |
Record name | Arsenic(V) acid, trisodium salt, heptahydrate (1:3:7) | |
Source | ChemIDplus | |
URL | https://pubchem.ncbi.nlm.nih.gov/substance/?source=chemidplus&sourceid=0064070833 | |
Description | ChemIDplus is a free, web search system that provides access to the structure and nomenclature authority files used for the identification of chemical substances cited in National Library of Medicine (NLM) databases, including the TOXNET system. | |
Record name | Arsenic acid (H3AsO4), sodium salt (1:3) | |
Source | EPA Chemicals under the TSCA | |
URL | https://www.epa.gov/chemicals-under-tsca | |
Description | EPA Chemicals under the Toxic Substances Control Act (TSCA) collection contains information on chemicals and their regulations under TSCA, including non-confidential content from the TSCA Chemical Substance Inventory and Chemical Data Reporting. | |
Record name | Trisodium arsenate | |
Source | EPA DSSTox | |
URL | https://comptox.epa.gov/dashboard/DTXSID9039367 | |
Description | DSSTox provides a high quality public chemistry resource for supporting improved predictive toxicology. | |
Record name | Trisodium arsenate | |
Source | European Chemicals Agency (ECHA) | |
URL | https://echa.europa.eu/substance-information/-/substanceinfo/100.033.334 | |
Description | The European Chemicals Agency (ECHA) is an agency of the European Union which is the driving force among regulatory authorities in implementing the EU's groundbreaking chemicals legislation for the benefit of human health and the environment as well as for innovation and competitiveness. | |
Explanation | Use of the information, documents and data from the ECHA website is subject to the terms and conditions of this Legal Notice, and subject to other binding limitations provided for under applicable law, the information, documents and data made available on the ECHA website may be reproduced, distributed and/or used, totally or in part, for non-commercial purposes provided that ECHA is acknowledged as the source: "Source: European Chemicals Agency, http://echa.europa.eu/". Such acknowledgement must be included in each copy of the material. ECHA permits and encourages organisations and individuals to create links to the ECHA website under the following cumulative conditions: Links can only be made to webpages that provide a link to the Legal Notice page. | |
Record name | TRISODIUM ARSENATE | |
Source | FDA Global Substance Registration System (GSRS) | |
URL | https://gsrs.ncats.nih.gov/ginas/app/beta/substances/7XO134LHLN | |
Description | The FDA Global Substance Registration System (GSRS) enables the efficient and accurate exchange of information on what substances are in regulated products. Instead of relying on names, which vary across regulatory domains, countries, and regions, the GSRS knowledge base makes it possible for substances to be defined by standardized, scientific descriptions. | |
Explanation | Unless otherwise noted, the contents of the FDA website (www.fda.gov), both text and graphics, are not copyrighted. They are in the public domain and may be republished, reprinted and otherwise used freely by anyone without the need to obtain permission from FDA. Credit to the U.S. Food and Drug Administration as the source is appreciated but not required. | |
Disclaimer and Information on In-Vitro Research Products
Please be aware that all articles and product information presented on BenchChem are intended solely for informational purposes. The products available for purchase on BenchChem are specifically designed for in-vitro studies, which are conducted outside of living organisms. In-vitro studies, derived from the Latin term "in glass," involve experiments performed in controlled laboratory settings using cells or tissues. It is important to note that these products are not categorized as medicines or drugs, and they have not received approval from the FDA for the prevention, treatment, or cure of any medical condition, ailment, or disease. We must emphasize that any form of bodily introduction of these products into humans or animals is strictly prohibited by law. It is essential to adhere to these guidelines to ensure compliance with legal and ethical standards in research and experimentation.